Use of optimisation modelling to inform water strategy: a case study
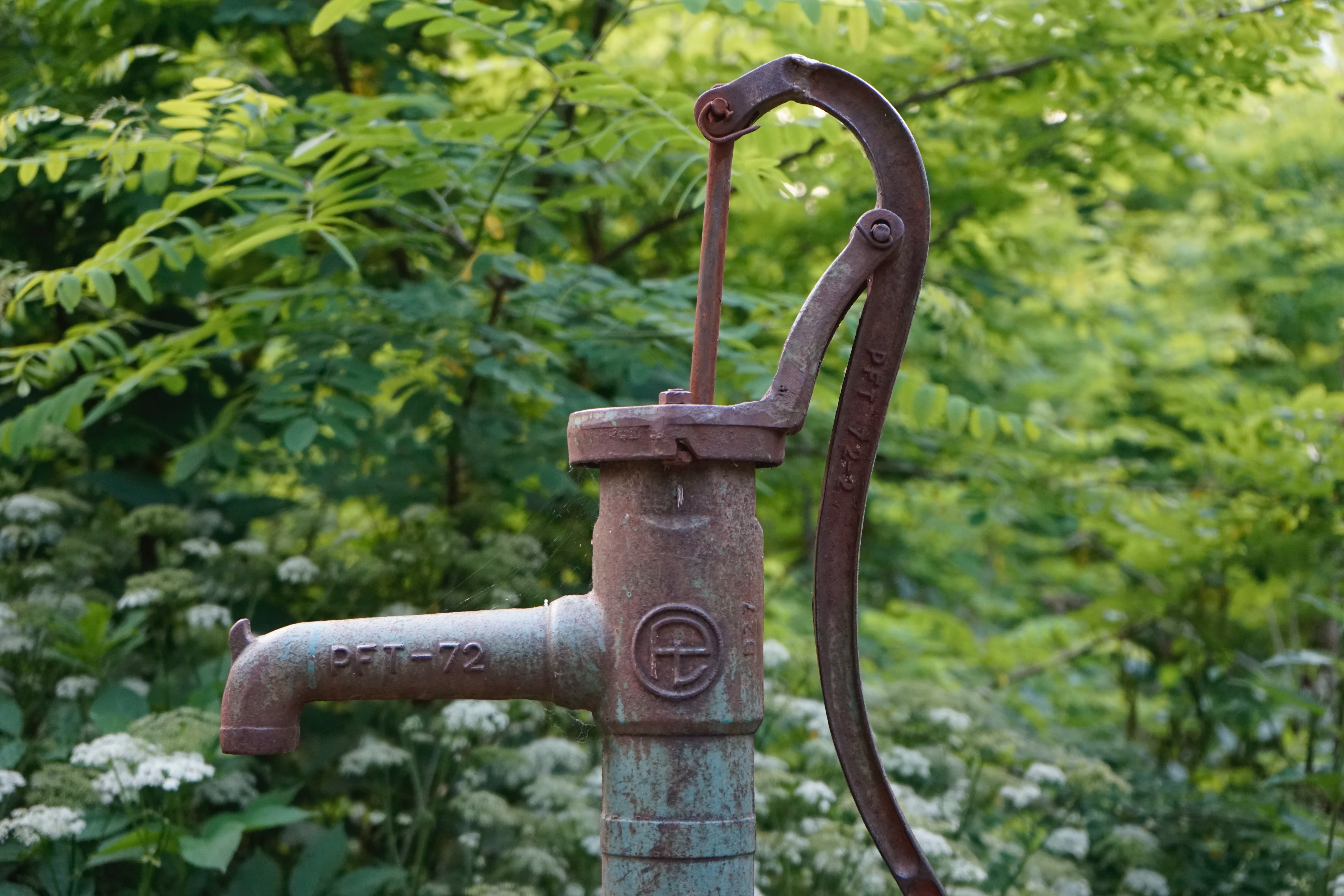
First published in Water e-Journal Vol 8 No 2 2022.
Optimisation modelling was applied to understand the potential groundwater shortfall in Perth’s urbanising northeast
DOWNLOAD THE PAPER
Abstract
Western Australia’s Perth and Peel regions are growing rapidly, with a population of over 2 million in 2016 projected to grow to 3.5 million people by 2050. Traditionally the green spaces in urban areas have been irrigated with groundwater. However, as Perth grows, urban development is increasingly moving into areas with limited groundwater availability.
This paper presents a case study in the northeast of Perth, where optimisation modelling was applied to understand the potential groundwater shortfall in an area undergoing urbanisation. The optimisation objective was to minimise the total gap between water supply and demand across all subareas (units of groundwater management) through a different selection of groundwater sources within the sub-areas and aquifers available to each development. Applying this model to a range of urban design and planning scenarios, we were able to identify critical factors influencing the potential water supply-demand gap. From this, strategies were developed to guide planning and reduce the likelihood that additional water sources will be required.
This is the first time we are aware that an optimisation modelling approach has been applied to groundwater planning in Western Australia. Current business-as-usual is for trades and transfer of groundwater licences (movement of a water licence from one party to another, taken from a different location or the same location, respectively) to progress on an ad-hoc basis, without detailed scenario planning. The key benefit of this approach is that it allows local government to examine the relationship between urban liveability, planning decisions, local planning and design policy, and other factors that will trigger the need for additional water sources.
Introduction
Western Australia’s Perth and Peel regions are growing rapidly, with a population of over 2 million in 2016 expected to grow to 3.5 million people by 2050 (Western Australian Planning Commission 2018). Traditionally these green spaces in Perth have been irrigated with groundwater. This has allowed a high standard of green space amenity at low cost, despite Perth’s dry summers.
With growth, urban development is moving into more water-constrained areas. This includes areas with lower groundwater availability for the irrigation of green spaces, as well as areas that are seasonally inundated due to shallow (but limited) groundwater.
There is a need for high-quality green spaces as a component of urban liveability, for the physical and mental health and wellbeing of communities, and for the potential to combat rising temperatures in urban areas (Government of Western Australia 2019, Productivity Commission 2020). Water availability is key in providing these green spaces.
Site details
Perth’s northeast corridor will accommodate a significant proportion of the demand for new dwellings and associated infrastructure within the metropolitan area. From an estimated 76,550 dwellings in 2011, the corridor is projected to contain 129,110 dwellings by 2050 (Western Australian Planning Commission 2018).
Within the northeast corridor, the Swan urban growth corridor (the study area) is the primary residential growth area. This corridor includes existing urban areas and developments under construction, as well as rural and agricultural (including horticulture) areas that have been designated for urban development. It will include some higher-density developments linked to a new train line. The corridor will contain regional open space and possibly additional sporting and recreation facilities. It is expected to be fully urbanised by 2040.
The study area lies within the Gnangara groundwater system. This is Perth’s largest, most accessible and lowest-cost source of drinking water. Parts of the study area are within the Gnangara public drinking water source area, where water quality protection measures influence planning decisions. The Gnangara system is under stress because of reduced rainfall due to climate change and continued groundwater abstraction. This has resulted in declining groundwater levels and negative impacts on wetlands and other groundwater-dependent ecosystems.
A new Gnangara groundwater allocation plan (Department of Water and Environmental Regulation 2022) has recently been released, with revised water allocation limits. Within the study area, all except one of the groundwater subareas (units of groundwater management) are fully allocated or over-allocated, so additional groundwater abstraction is not supported.
The study area is shown in Figure 1. It shows that some areas are undeveloped, and partially or fully developed. The two potential locations for the regional open space are shown.
Figure 1. Study area showing the potential locations of the regional open space (approximate location shown by red dot; Location 1 in the south, and Location 2 in the east)
Project aims
Given the potential groundwater shortfall in this area, this study was undertaken to understand water supply and demand, and to evaluate the need for additional water sources.
If additional water sources are needed, such as treated wastewater or drinking water, they are considerably more expensive and/or complex than groundwater. Assessment is needed to ensure that any additional water source is appropriate, efficient and affordable.
This work therefore aimed to:
- Develop a water supply-demand model for the study area using optimisation modelling to minimise the groundwater shortfall
- Apply the model to a range of urban design and planning scenarios to understand the critical factors impacting the potential for groundwater shortfall
- Develop strategies to guide further planning and reduce the likelihood that additional water sources will be required
Modelling approach
Optimisation modelling is used in a range of fields to find the best available values of an objective function in a defined domain. It is commonly used to inform decision-making on the optimal use of resources and has been applied to water planning purposes such as minimising the total cost of supplying water (Abdulbaki et al 2017), identifying optimal allocation (Kondili et al 2010), planning optimal desalination (Shahabi et at 2017), and managing river basins (Wu et al 2015).
Wu et al (2017) applied optimisation to integrated urban water management to inform policy aimed at prioritising the development of different water resources in Adelaide. An optimisation-based approach considered traditional water sources (surface water from reservoirs and rivers) and alternative water sources (such as desalinated seawater, harvested stormwater, and reclaimed wastewater). Multi-objective optimisation considered economic cost and energy, along with considerations such as reliability, and the environmental impact of stormwater and wastewater discharge on receiving waters, to propose an optimal mix of sources. More recently Chakraei et al (2021) developed an integrated simulation-optimisation model that can be used to optimise decisions including the operation of a reservoir, allocations of water to irrigation canals, and abstraction of water from river and groundwater sources.
In this work, a simplified approach was applied. Groundwater in Western Australia is managed through water allocation limits, which are informed by the Perth Regional Aquifer Model (PRAMS), a coupled saturated/unsaturated flow model. Groundwater can be licenced up to the allocation limit of the groundwater subarea. Groundwater licences allow the user to take water to a set volumetric limit, and in this study area, licenced groundwater entitlements are generally fully used.
Given the annual time step for licenced allocations in the study area, and full use of licenced water volumes, we were able to consider future supply based primarily on the trade and transfer (movement of a water licence from one party to another, taken from a different location or the same location, respectively) of existing licences. Current business-as-usual in a fully allocated sub-area with changing land use is that this would occur on an ad-hoc basis.
The approach undertaken in this work was to model future supply (informed by PRAMs and water allocation planning), future demand (informed by land planning and urban design decisions), and consider the future groundwater shortfall, using optimisation for the trade and transfer of existing licences. This type of supply-demand scenario modelling is a common approach to water supply planning, for example, as used in the ‘Western trade coast heavy industry local water supply strategy’ (Department of Water 2016), however, we are not aware of optimisation modelling having been applied in this context previously.
Method
The overarching approach to modelling was to:
- Understand the existing groundwater situation comprising licensed groundwater entitlements (including those that will become available with land use change) and available groundwater volumes.
- Understand the potential future water demand (primarily for the irrigation of green spaces in urban development) and how this may vary with urban and green space design and planning decisions.
- Use the above to understand the potential for a groundwater shortfall (water supply-demand gap).
The building blocks for the model are groundwater subareas. These are administrative units used in water resources planning and the regulation of groundwater licences in Western Australia. Each subarea usually has multiple aquifers, both superficial and confined. An allocation limit is set for each aquifer within each subarea, and groundwater can be licenced for abstraction up to the allocation limit.
A person whose property is located within a subarea may apply for a licence to access groundwater in that subarea. Where a planned urban development extends across the boundary between two groundwater subareas, developers could potentially access groundwater from aquifers in either or both subareas, if available. We sought to model how future urban developments could most efficiently access groundwater, taking into account subarea and aquifer constraints.
Model Inputs
Groundwater availability with land use
As urban development proceeds, existing rural and agricultural landowners will depart the study area. It is expected that they will trade or transfer their water licenses to developers or the local government.
To understand groundwater availability, we examined all water licences within the study area and identified:
- licences held by licensees that are expected to remain after the development (such as existing local government and school licences for irrigation)
- licences held by developers, which will be transferred to the local government on handover of the development
- short-term licences (e.g. for dust suppression and turf establishment) that cannot be renewed, or transferred to new licensees.
The remaining groundwater licences were considered likely to become available for trade or transfer, subject to an assumed 10% reduction in groundwater licences by 2030 (to account for the Gnangara Groundwater Allocation Plan (2022) required 10% reduction between 2028 and 2032). Additionally, any unused groundwater licences were assumed to be recouped by the Department, as required by the Plan.
Water demand for green spaces
The requirements for the provision of public open space are set in Liveable Neighbourhoods (Western Australian Planning Commission 2009). In residential areas, a minimum of 10% of the gross subdivisible land area must be provided by the developer for public open space. Developers are responsible for designing and constructing these spaces and securing a water source that is transferred to the local government at the handover of the development.
Historically the Department has allocated groundwater for public open space using an irrigation rate of 7500kL/ha/y. Given the Gnangara Groundwater Allocation Plan (2022) 10% reduction in allocations, this rate has been reduced by 10%, to 6750kL/ha/y for this area.
For the purposes of this modelling, two demand scenarios were evaluated:
Base demand
The base demand is intended to be an estimate of the volume of water required to irrigate public open space to an acceptable standard and maintain liveability. It is intended to allow for district and regional open spaces (playing fields) to be fully irrigated to maintain their functionality. Other grassed or planted areas can be maintained through summer by irrigation, depending on design choices.
An irrigation rate of 6750kL/ha/y was assumed for 60% of the public open space area; with 40% of public open space assumed to be unirrigated. This unirrigated area could be native vegetation or alternative uses such as playgrounds. This is similar to the irrigation regime developed and applied in the North west corridor water supply strategy (Department of Water 2014). Waterwise design with hydrozoning (grouping types of vegetation into categories with similar water requirements to reduce overwatering) and ecozoning (areas of native garden areas rather than underutilised turf) would be required to achieve this rate of water use.
Base demand was calculated based on the following assumptions:
- Public open space will be 10% of the gross sub-divisible area of a development (which is 80% of the total development area).
- Water use is assumed to be equivalent to irrigating 60% of the public open space area at an irrigation rate of 6,750 kL/ha/year.
- Additional demand for district and regional open space as provided by the local government.
- Irrigation water is not required for other non-residential land, including street verges, median strips, entry statements and private green spaces.
High demand
A high demand scenario was modelled as an upper bound for water demand in the study area. This scenario was calculated assuming 20% public open space, with our other assumptions unchanged.
This scenario is also indicative of water demand with a lower amount of public open space, and a higher rate of water use (through a higher irrigate rate or higher proportion of public open space being irrigated). As such, it provides an indication of the impacts of green space design choices on water use.
Estimated water demand for the low and high demand scenarios is shown in Figure 2. Note that the location of the regional open space (red dot) is still under consideration and may alternatively proceed as shown in Figure 1. Some of the areas shown are partly developed; in these cases estimated demand is in addition to current groundwater usage.
Figure 2. Estimated additional water demand under the low demand scenario (left) and the high demand scenario (right)
Modelling process
The model was designed to minimise the total supply-demand gap across all groundwater subareas through selection of different water sources (aquifers) within the subareas available to each development. Optimisation was for the planned urban developments and open spaces at full development.
The modelling process consisted of three steps:
- Develop a water demand forecast for the study area comprising:
- Demand from users that will remain within the development footprint post-urbanisation (i.e. the local government, schools, and some of the developer licences) to 2040
- Future public open space demand to 2040
- Future regional and district open space demand to 2040
- Develop a spatial and temporal groundwater availability forecast for the study area to 2040 including:
- Current groundwater licences that remain post-urbanisation (i.e. the local government, schools and some of the developer licences)
- Groundwater licences that are expected to become available for trade or transfer (subject to recouping unused licences or part-licences)
- Groundwater licences currently issued to developers for irrigation
- Available groundwater allocations (in the Beechboro subarea)
- Develop the linear programming optimisation model and use the results of the previous two steps to obtain model parameters and identify constraints.
For the optimisation model, the objective function is the minimum total water supply-demand gap across all subareas. The decision variables are the volume of water to be abstracted from each groundwater subarea and aquifer. The constraints are the allocation limits of the subareas and aquifers.
The study area was divided into 22 sections (17 new developments and 5 existing developments). The water sources for the study area are current groundwater licences, licence trades and transfers, and available allocations. The number of decision variables is 66 (22 sections x 3 sources).
The model was developed in Microsoft Excel and run using the solving method Simplex linear programming.
Scenario development
Modelling scenarios were designed to understand the potential for a groundwater shortfall under a range of urban design and planning assumptions. These considered:
- The impact of urban design on the potential for a groundwater shortfall. This was done by comparing:
- baseline vs high demand with regional open space in Location 1 (Scenario 1 vs Scenario 3), and
- baseline vs high demand with regional open space in Location 2 (Scenario 2 vs Scenario 4).
- The impact of planning decisions. We evaluated the impact of the location of the regional open space (as shown in Figure 1) through comparing:
- Location 1 vs Location 2 with baseline demand (Scenarios 1 vs Scenario 2), and
- Location 1 vs Location 2 with high demand (Scenario 3 with 4)
- The impact of ongoing climate change through an additional 10% in groundwater abstraction in 2040 (comparing Scenario 3 with Scenario 5). This is not being considered as a policy change but provides useful information about the sensitivity of the groundwater shortfall to climate change impacts.
The scenario assumptions are summarised in Table 1.
|
Water supply |
Water demand |
|||||
Scenario |
10% reduction in 2030 |
10% reduction in 2040 |
All trades proceed |
10% reduction in abstraction |
Baseline demand: 10% public open space in new developments |
High demand: 20% public open space in new developments |
Regional open space location |
1 |
√ |
|
√ |
√ |
√ |
|
Location 1 |
2 |
√ |
|
√ |
√ |
√ |
|
Location 2 |
3 |
√ |
|
√ |
√ |
|
√ |
Location 1 |
4 |
√ |
|
√ |
√ |
|
√ |
Location 2 |
5 |
√ |
√ |
√ |
√ |
|
√ |
Location 1 |
Results
Model output
The model outputs are the optimal groundwater abstraction regime by development (i.e. how much water can be accessed from which available subarea and aquifer in each development footprint), and the total water supply-demand gap (or surplus) by subarea.
This is summarised in Table 2 and shown for Scenarios 1 and 3 in Figure 3. In Figure 3, ‘trade and transfer risk’ areas are identified. These are areas where there could be a groundwater shortfall if the expected trades and transfers do not proceed (for example, if a departing land user trades water to a party outside the study area).
Considering the model outputs by development, it was also possible to evaluate water supply constraints and options by development for each scenario.
Note that while there is some surplus groundwater under each scenario, this water is not in the groundwater subareas where there is the demand for water.
|
New development demand (ML) |
Existing development demand (ML) |
Optimum supply |
Gap (ML) |
Surplus (ML) |
||
Available entitlement (ML) |
Existing entitlement (ML) |
Trades (ML) |
|||||
1 |
579 |
2,277 |
394 |
2,277 |
78 |
107 |
478 |
2 |
612 |
2,277 |
131 |
2,277 |
78 |
402 |
741 |
3 |
964 |
2,277 |
517 |
2,277 |
207 |
240 |
161 |
4 |
1,029 |
2,277 |
287 |
2,277 |
207 |
535 |
391 |
5 |
983 |
2,277 |
523 |
2,050 |
202 |
487 |
107 |
Figure 3. Estimated groundwater shortfall (mL/year) for scenario 1 (left) and scenario 3 (right)
Discussion
This modelling provides information on the availability of groundwater to support development under a range of scenarios. Based on the results, the critical factors influencing the supply-demand gap are:
- The quantity of water that developers seek for the irrigation of green spaces in new developments and for sports facilities (related to the area of green spaces).
- Decisions about future land use within the study area. This includes decisions about the location of the regional open space (and potential additional sports facilities), and whether development areas currently under investigation proceed to be developed.
Through consideration of the model results, we propose four strategies seeking to ensure that additional supplies are only developed if needed, balanced with maintaining future supply options and avoiding fragmented outcomes.
Strategy 1: Set local government priorities and preferences
The modelling results show that the area of irrigated public open space has a large impact on the potential groundwater shortfall. For example, comparing the baseline and high demand scenarios (Scenarios 1 and 3; comparing 10% public open space with 20% public open space across the study area, with the regional open space in Location 1) the estimated groundwater shortfall increases from around 100 ML to 240 ML/year.
The baseline scenario depicts a rate of irrigation that requires waterwise design, with careful use of water. While the high demand scenario was developed based on a larger area of public open space area, the findings are similar for an unchanged area of public open space with higher water usage, indicating that demand can be influenced by both design criteria and standards for green spaces.
Local government, as the final custodian of urban developments, has a leading role in influencing the form and design of new developments and irrigated spaces, and setting preferences for new water sources. Through local planning strategies, schemes and policies, local governments can establish the planning context for their area and implement the local planning strategy (Western Australian Planning Commission 2014).
There is a role for local governments to consider their objectives for green spaces, balancing water management implications with the importance of green spaces in providing liveability in a drying climate, and taking into account State Government policy, housing density and community needs. It may be appropriate that the provision of green spaces be varied between areas or developments. However, this should be done in a considered manner regarding the implications for water management, and potentially triggering the need for additional sources.
Strategy 2: Optimise use of groundwater within allocation limits
Modelling demonstrates that with baseline water demand there is potential for groundwater to supply all the water required with the exclusion of one development area, as shown in Figure 3. There is a trade and transfer risk in some areas, so avoiding the need for additional water will require careful and orderly use of groundwater.
To make the best use of groundwater:
- Local government should set out its requirements for green spaces in new developments, including the area of green space, design criteria and irrigation standards, in alignment with Strategy 1. Landscaping and the design of new developments should apply water sensitive urban design principles, including non-irrigated green spaces. Particularly in groundwater-constrained areas, there is a need to ensure new and existing green spaces are waterwise, balancing reduced water use with liveability outcomes. In this study area, modelling has demonstrated that waterwise design and efficient use in new developments will be critical in determining the realised magnitude of the supply shortfall (through comparison of the baseline water demand with the high water demand scenarios).
- Developers and the local government should acquire groundwater licences from current licence holders as these become available with land use change.
- Local government should investigate the strategic redistribution of water from areas that are already developed. There is scope to increase water use efficiency in developed areas and redistribute groundwater licences to new developments within the same groundwater subarea.
Strategy 3: Identify development-scale water source options
The modelling results show that there is one development that will require additional water if it proceeds (Figure 3, area shown in orange and red), under all scenarios. Some developments may need an additional source, dependent on water licence trades and transfers proceeding. Particularly for developments identified as ‘trade and transfer risk’ (Figure 3), should developers seek a higher level of irrigation water than assumed in modelling, additional water will be required. This should be investigated early in the planning process.
In general, the triggers for an additional water source are:
- urban development proceeding in areas where it is not possible to secure sufficient groundwater for the planned area of green space under the ‘baseline demand’ scenario.
- a desire for developments to have a higher level of amenity through more green spaces than possible with groundwater use alone. This will, in part, depend on how the available groundwater is shared between developments.
- the developer chooses not to use existing groundwater or to pursue trades or transfers to meet their water needs.
Davies et al (2016) identified the use of sub-surface drainage as a potential water source in this area. In areas of shallow groundwater, this drainage is installed to manage groundwater levels and prevent damage to infrastructure. This is currently under investigation as an option within the study area.
Strategy 4: Maintain options for corridor-scale water sources
The impact of future climate change is illustrated through a comparison of Scenario 5 with Scenario 3. Scenario 5 models a 10% reduction in water availability by 2030, with an additional 10% reduction by 2040 to provide an indication of the sensitivity of the groundwater shortfall to climate change impacts. This was developed as a worst-case scenario. It results in a groundwater shortfall that significantly exceeds any surplus in the study area.
Given that there could be a future shortfall in the long term, along with potential future demand for water beyond the study area, local government should seek to maintain water source options in the study area. While a corridor-scale solution appears unlikely to be warranted before 2040, given the scale of sub-surface drainage required in the study area plus climate change effects, harvesting of this water may present a future opportunity.
Where feasible, new developments should maintain the option of future harvesting of drainage water. This could be done through the design of drainage systems to centralised hubs, subject to the assessment of any regional-scale environmental impacts. In designing this, the primary function of drainage systems to protect infrastructure should not be compromised to provide water for harvesting, nor should environmental systems be adversely impacted. This may require coordination across developments and can be influenced by local government land use planning.
Benefits of this approach
Detailed water planning is not generally undertaken at the sub-regional scale in this way. However, with a drying climate and reduced availability of groundwater for green spaces, there may be an increased need for such planning.
In this study, we have applied a new approach to sub-regional planning using an optimisation model. This was more resource-intensive to initiate than a manual approach, given the need to develop the modelling first. However, this was offset by the benefits:
- This approach allowed for the investigation of many scenarios and planning decisions (beyond those reported in this paper). Modelling assumptions can easily be changed, for example, it is possible to change variables (such as irrigation rates, or the percentage of possible trades and transfers that proceed) and understand the impact of these on the supply-demand gap.
- The model can be used to investigate policy and planning changes. It allowed us to work with stakeholders and test model assumptions or update understanding with stakeholders in real-time.
- Through scenario modelling, it was possible to identify the critical factors that influence a groundwater shortfall and use these to inform planning strategies for local government.
- As decisions are made, or further information becomes available, the model can be run to provide updated scenario predictions.
As planning decisions are resolved, the model could be expanded to develop an optimal schedule of licences that could be used to achieve the best water supply outcome in the study area.
Conclusions
This case study applied optimisation modelling to understand the potential groundwater shortfall in an area undergoing urbanisation.
The optimisation objective was to minimise the total gap between water supply and demand across all subareas (units of groundwater management) through the different selection of groundwater sources within the subareas and associated aquifers available to each development.
Applying this model to a range of urban design and planning scenarios allowed us to identify the critical factors influencing the potential water supply-demand gap:
- The quantity of water that developers seek for the irrigation of green spaces in new developments and for sports facilities.
- Decisions about future land use within the study area. This includes decisions about the location of the regional open space (and potential additional sports facilities), and whether development areas currently under investigation proceed to be developed.
On this basis, we propose four strategies seeking to ensure that additional water sources are only developed if needed, balanced with maintaining future water source options and avoiding fragmented outcomes or missed opportunities. If implemented, these strategies will guide land use and water planning, and reduce the likelihood that additional water sources will be required in the study area.
We have shown that careful design and efficient use of local groundwater resources will help to avoid the need for additional water sources (depending on planning decisions) while maintaining liveability. Optimising the use of groundwater could limit the need for more expensive and complex water sources.
Acknowledgements
The authors acknowledge the contributions from state and local government towards this work, and the comments provided by anonymous reviewers, which improved the manuscript.
References
Abdulbaki, Dunia, Al-Hindi Mahmoud, Yassine Ali A., Abou Najm, Majdi. 2017. “An optimisation model for the allocation of water resources”. Journal of Cleaner Production 164.
https://doi.org/10.1016/j.jclepro.2017.07.024
Beh Eva H Y., Dandy G C., Maier H R., Paton F. L (2014) Optimal sequencing of water supply options at the regional scale incorporating alternative water supply sources and multiple objectives, Environmental Modelling and Software 53:137–153.
10.1016/j.envsoft.2013.11.004
Chakraei, I, Safavi, H R, Dandy, G C, Golmohammadi, M H (2021) Integrated simulation optimization framework for water allocation based on sustainability of surface water and groundwater resources, Journal of Water Resources Planning and Management, 147 (3)
https://doi.org/10.1061/(ASCE)WR.1943-5452.0001339
Davies, Carl G., Vogwill, R., and Oldham. C. 2016. “Urban subsurface drainage as an alternative water source in a drying climate” Australasian Journal of Water Resources 20(2):148-159. https://doi.org/10.1080/13241583.2017.1351130
Department of Water. 2014. North west corridor water supply strategy.
Department of Water and Environmental Regulation. 2022. Gnangara groundwater allocation plan.
Government of Western Australia. 2019. Waterwise Perth Action Plan. Perth.
Kondilia E., Kaldellis, J.K., Papapostolou C 2010. A novel systemic approach to water resources optimisation in areas with limited water resources, Desalination 250(1) 297-301
https://doi.org/10.1016/j.desal.2009.09.046
Productivity Commission. 2020. Integrated urban water management – Why a good idea seems hard to implement. Canberra.
Shahabi M. P., McHugh A., Anda M., Ho G. 2017. A framework for planning sustainable seawater desalination supply. Science of the total environment 575:826-835. https://doi.org/10.1016/j.scitotenv.2016.09.136
Western Australian Planning Commission, 2009. Liveable Neighbourhoods. Perth. https://www.wa.gov.au/government/publications/liveable-neighbourhoods
Western Australian Planning Commission. 2018. Perth and Peel @ 3.5 million frameworks. https://www.wa.gov.au/government/publications/liveable-neighbourhoods
Wu B, Zheng Y,Xin W,Tian Y,Han F, Liu J, Zheng C, 2015. “Optimizing water resources management in large river basins with integrated surface water-groundwater modeling: A surrogate-based approach” Water Resources Research 51(4):2153-2173.
https://doi.org/10.1002/2014WR016653
Wu, W, Dandy, G C, Maier, H R, Maheepala, S, Marchi, A and Mirza, F (2017) Identification of optimal water supply portfolios for a major city, Journal of Water Resources Planning and Management, 143 (9)
10.1061/(ASCE)WR.1943-5452.0000811
Author Biographies
Melissa Bromly | Melissa is Senior Water Planner (Water Supply Planning) in the Western Australia Department of Water and Environmental Regulation. She has degrees in environmental engineering and soil science, and a PhD in modelling solute transport from the University of Western Australia.
Maedeh Shahabi | Mae has been a Water Supply Engineer in Western Australia Department of Water and Environmental Regulation, a since 2015. She has PhD in engineering from Murdoch University. Mae’s work and research experiences comprise developing quantitative frameworks and tools for sustainable water supply planning.
Matthew Awang | Matthew is the Manager of Water Supply Planning in the Western Australia Department of Water and Environmental Regulation. He has a Master of Science in Hydrogeology and Groundwater Chemistry from the University of Reading.