PFAS in biosolids: A review of international regulations
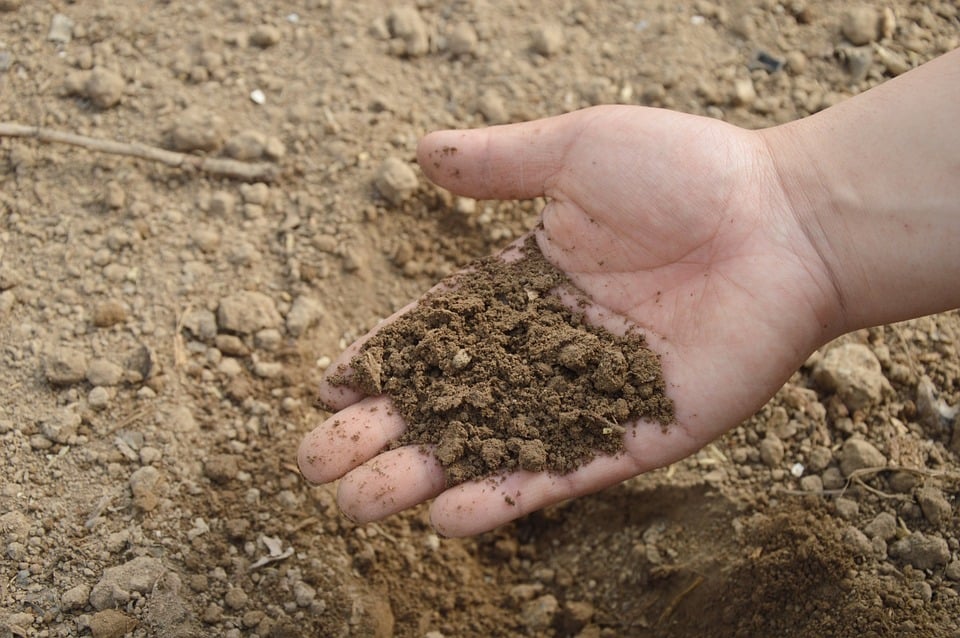
First published in Water e-Journal Vol 5 No 4 2020.
Insights into global PFAS management and the Australian context
DOWNLOAD THE PAPER
Abstract
Per- and polyfluoroalkyl substances (PFAS) are incredibly useful additives, often providing excellent surface tension-lowering properties to a material. Due to the extensive use of PFAS in daily life in developed countries, PFAS invariably collects in municipal wastewater. Without targeted removal of PFAS at wastewater treatment plants, PFAS can move through the treatment process into both the recycled water and biosolids.
The presence of PFAS in biosolids poses a potential challenging problem to society for many reasons. A small number of countries have cautiously started, or have at least considered, limiting the concentration of PFAS permitted in biosolids that are to be used for land application.
Our review covers the current limits on PFAS concentrations in Australian biosolids, along with the latest developments in international regulations. We found that only Maine, USA, has set upper limits of PFAS for “beneficial use of solid wastes”. Denmark, Germany, The Netherlands, Sweden and Australia have set PFAS limits in soils. No other countries were found to have PFAS limits relating to biosolids or their use; however, this also reflects the lack of industrialisation and centralised wastewater management in many parts of the world.
Introduction
Per- and polyfluoroalkyl substances (PFAS) is used as the collective noun for this group of chemicals, typically characterised by a hydrophilic carboxylic acid or sulfonate head and fluorine-saturated, hydrophobic tail. They are incredibly useful additives, often providing excellent surface tension-lowering properties to a material. It is because of their usefulness that they are now so ubiquitous in the environment, particularly in developed countries.
There has been international interest in PFAS and their impacts on the environment and human health since the 1990s. Key sites of concern have been factories that manufacture (or have manufactured) PFAS, airports and military bases. At these sites, PFAS have contaminated surrounding soil and from there they have leached into groundwater. Major groundwater contamination has occurred in this manner in many developed countries including the US, Germany, The Netherlands, Sweden, Canada and Australia, and is likely to be present in many more.
Although international regulation via the Stockholm Convention has reduced the use of perfluorooctane sulfonate (PFOS) and perfluorooctanoic acid (PFOA) specifically, not all uses for these chemicals have been restricted. The reason for the exempt uses is primarily because no chemical substitutes are readily available and the benefit of using PFAS outweighs the chronic risk. For example, PFAS surfactants are added to metal plating baths to protect workers from hexavalent chromium vapours.
However, as there are at least 4,700 PFAS currently available (OECD, 2018), the Stockholm Convention restrictions for PFOS and PFOA mean that there are still many more PFAS that can be used. Additionally, not all countries abide by the Stockholm Convention and therefore may continue to use PFOS and PFOA. Nevertheless, many countries do, and there is direct evidence that PFOS and PFOA concentrations in wastewater have significantly reduced since these chemicals were listed (Ulrich et al, 2016).
With growing concern over the abundant uses of PFAS, governments and researchers have sought to identify key sources of PFAS in the environment. At this point, land-applied biosolids have become of interest. Due to the extensive use of PFAS in daily life in developed countries, PFAS invariably collects in municipal wastewater. Without targeted treatment of PFAS at wastewater treatment plants, PFAS can move through the treatment process into both the recycled water and biosolids, noting that there is currently no readily available technology to remove PFAS from biosolids.
In Australia, land application is the most common use of biosolids from wastewater treatment plants. This includes spreading on agricultural land, biosolids that are composted prior to landscaping and horticultural use and land rehabilitation sites such as mines. Of the 371,000 dry tonnes (or 2.3 million wet tonnes) of biosolids produced in Australia in 2018/19, 67% were applied to land and 24% used for other beneficial uses such as composting and land rehabilitation (PSD, 2019).
Few countries have started taking steps to limit PFAS concentrations in biosolids, with many countries throughout the developing world still struggling to implement centralised sanitation schemes, let alone produce a biosolids product fit for regulated land application.
The purpose of this paper is to summarise the latest developments in international PFAS limits in biosolids and compare them with Australian limits.
PFAS and biosolids
As of September 2020, only 40 studies of PFAS concentration in biosolids had been published globally. Given the vast number of PFAS that are currently in use and the variability in wastewater catchments, our understanding of PFAS contamination in biosolids internationally is in its infancy. However, the majority of these studies found detectable levels of PFAS.
Understanding the relative concentration of PFAS in various materials provides some perspective on our everyday level of exposure to these chemicals. Table 1 provides a selection of published PFAS concentrations detected in a range of everyday products used in developed countries. The materials included in the table also demonstrate sources of PFAS in wastewater. Cosmetics, detergents, sunscreen and dust can all be washed down the drain, entering the sewer system and ultimately ending up in biosolids.
Table 2 provides a summary of published PFAS concentrations in biosolids based on geographic location for comparison with the data provided in Table 1.
Note that PFAS concentrations in research papers are usually published in ng/g, which is equivalent to 1 part per billion (ppb).
As long as PFAS continue to be used by industry and are present in consumer products, they will very likely be found in biosolids.
The presence of PFAS in biosolids poses a potentially challenging problem to society for some of the following reasons:
- PFAS source control is difficult due to the ubiquity PFAS in modern life.
- Due to the strength of the carbon-fluorine bonds PFAS are more likely to stay in the environment, rather than breakdown.
- Biosolids are a bulky material produced in large volumes and there is currently no practical way to concentrate the PFAS into a more manageable portion of material.
- There are few treatment options available for removing PFAS from biosolids.
- Incineration or pyrolysis process enables the thermal destruction of biosolids but with operational difficulties such as potential release of volatile fluorine and incomplete destruction, and limitations on recovery of organic matter or nutrients. Pyrolysis of biosolids is not currently a common practice and therefore significant new infrastructure would be required for this purpose. These processes also create a residual which would require management.
- Biosolids producers have already invested in treatment infrastructure to create a product primarily suitable for land application, prior to knowing of PFAS in biosolids.
A small number of countries have started, or at least considered, limiting the concentration of PFAS permitted in biosolids that are to be used for land application. The following sections summarise how this is being progressed in different parts of the world.


Australian situation
PFAS management in Australia has primarily been led by the Heads of EPAs Australia and New Zealand (HEPA) with the publication of the PFAS National Environmental Management Plan (NEMP) in 2018. A revised PFAS NEMP Version 2.0 was published in January 2020. Although biosolids do not have a specific upper limit for PFAS in this document, the NEMP Version 2.0 recommends that the ecological guideline values for soil should be considered when completing a risk assessment for the land application of biosolids. The soil values for all land uses are provided in Table 3. Specific guidance for biosolids and recycled water is yet to be released by HEPA; however, this is planned for in the NEMP work program.

Despite these guidelines, biosolids land application in Australia is governed by individual states and no state guidelines stipulate the NEMP ecological guideline values.
In addition to the NEMP, the Council of Australian Governments released a National PFAS Position Statement in February 2020. This document does not mention biosolids, however it does state that:
All Australian governments agree that further release of PFAS into the environment from ongoing use should be prevented where practicable, and that actions to reduce or phase out the use of PFAS should be nationally consistent.
Beyond this national framework, some tentative steps have been taken by the states to implement more prescriptive limits for biosolids. The Queensland End of Waste Code Biosolids, published in January 2020, offers the most biosolids-specific guidance, with trigger values for PFAS in soils, as shown in Table 4. Notification is required if any of the trigger values are exceeded following biosolids land application. It would therefore be prudent to know the PFAS contaminant concentrations in both the biosolids and soil prior to application.

EPA Victoria has an interim position statement (EPA Victoria, 2020), but this does not specifically provide an upper limit for PFAS in biosolids. However, there is an interim criteria for reuse of PFAS-impacted soil and this is based on a limit of reporting of 0.004 mg/kg (equal to 4 ng/g) from the sum of PFOS, PFHxS and PFOA.
Apart from these guidance documents, all other states and territories defer to the NEMP Version 2.0 and therefore currently lack specific limits for PFAS in biosolids.
International regulations and research
Europe
As of September 2020, no European countries were identified which had introduced specific rules or limitations around PFAS concentrations in biosolids for land application, with the exception of several German Lander (states). What is striking is the variety of approaches to management of PFAS across Europe both in terms of if regulations are in place and how those regulations are set. Table 5 summarises the approach of European countries that have set PFAS limits in soil and fertiliser. Denmark, Germany, The Netherlands and Sweden have set national limits for PFAS in soil. Germany has set a limit for PFAS in fertiliser, which also applies to biosolids used as a fertiliser.

For the countries that have set soil limits, we note the following:
- Denmark is a leader in PFAS regulation. It has developed a suite of actions including a planned ban of PFAS treated food contact materials in 2020.
- Germany has had well documented social and environmental problems with PFAS specifically linked to land application of sewage sludge contaminated with industrial wastes. This led to possibly the first PFAS regulation which was a limit of 100 micrograms PFC/kg (0.1 mg/kg) in several German regions (German Federal Environment Agency, 2009). It is unclear if this regulation still stands or if the nationwide limit applies.
- The Netherlands has taken a different approach of only limiting PFOS and PFOA under a temporary regulatory arrangement. The ubiquitous nature of PFAS in soils saw many dredging and construction works paused in 2019 causing some political pushback on these limits.
- Sweden’s Special Commission on PFAS has developed interim guidelines for sensitive land use (i.e. residential or gardens) and less sensitive land use (i.e. industrial or offices) based on the most sensitive receptor for that land use. The receptors considered are health based (oral intake soil, dermal exposure, dust inhalation, vapor inhalation, drinking water and plant intake), soil biota, groundwater protection and surface water protection. For the more sensitive land use the driving factor is soil biota. For the less sensitive land use, the driver is groundwater protection. This approach highlights the benefits to a risk-based approach to PFAS limits in the environment, which is the end goal of the Swedish regulations.
Several countries have not set limits but are using a suite of tools to reduce exposure such as source reduction, risk-based assessment of clean up proposals and development of strategies for assessing and addressing PFAS contamination. These include:
- Norway has taken the approach of communication with industry and the public, and strategic regulations are supported by risk assessment and cost benefit analysis. Some of these regulatory measures include clean-up of contaminated sites, and a ban on the manufacture, production, import and sale of consumer products containing PFOA. Annual PFAS analysis of treated effluent and the environment have shown a decreasing trend.
- Finland is undertaking a risk-based approach to PFAS contamination in soil or groundwater, promoting an approach that assesses the actual risk and remediation suitability of each situation. Hard limits will not be set.
- The United Kingdom Environment Agency is developing a strategy for sludge in the context of ongoing PFOS contamination which will review the use of sludge on agricultural land.
- Denmark, Germany, Norway, Sweden and The Netherlands are taking strong regulatory and practical action and are seeing PFAS concentrations drop across a range of monitored applications and in the environment. The approach is holistic and advocates source reduction, as well as end use limits.
Internet searches did not reveal any other European countries where PFAS limits relating to biosolids or land application were set or a specific strategy was in use, even in countries with well documented approaches to managing PFAS in groundwater, or where PFAS contamination has been extensive.
United States of America
The US EPA published a PFAS Action Plan in February 2019. As yet it has not set an upper limit for PFAS in biosolids as it has not completed a risk assessment of the impacts of PFOS and PFOA in land-applied biosolids. This risk assessment is due in 2020. The US EPA is also in the process of developing and validating testing methods to measure PFAS in complex matrices, including soils, biosolids and sediments. Validated testing methods are due in 2021.
Although PFAS contamination is widespread in the US, state-based PFAS limits have primarily been implemented for drinking water. In 2016, Vermont brought in a preliminary soil screening value of 0.3 mg/kg (300 ng/g) which is not specific to biosolids. Apart from this action taken in Vermont, Maine is the only state to have implemented a limit directly relating to solid waste for beneficial use.
PFAS has been an issue in Maine due to groundwater contamination close to former military installations. Subsequent to this discovery, more widespread groundwater contamination was identified, and further investigation showed PFAS in drinking water, milk, hay and soils, all of which had links to PFAS-contaminated groundwater.
The Maine Department of Environmental Protection created a PFAS Task Force in 2019 to investigate state-wide contamination of PFAS and make recommendations for action to reduce contamination and protect human health. The Task Force included specialists in wastewater treatment and biosolids management, and specifically addressed the contamination of wastewater residuals with PFAS. In January 2020 the Task Force published its final report recommending the following actions are taken regarding the beneficial use of wastewater residuals:
- Regular testing of all wastewater residuals for PFAS prior to land spreading or commercial distribution.
- Investigate the availability of treatment and disposal technologies that minimise the potential for environmental PFAS contamination.
- The state should promote the development of infrastructure, on the scale necessary to meet the needs of the state and to manage PFAS-contaminated wastes safely and in a cost-effective manner.
- Advocate for federal support to develop agronomic models of PFAS uptake from residual treated soils.
Chapter 418 of the Maine Solid Waste Management Rules (2018) details how solid wastes can be beneficially used. Appendix A of this document lists the screening levels for 165 contaminants in the solid wastes, with the values derived using the standard risk assessment protocols of the US EPA. That is, the screening levels are set at the lowest level of exposure, based on the exposure that is most likely at the beneficial use site. For PFAS, the screening levels are based on the leaching to groundwater pathway and are set at:
- Perfluorobutane sulfonic acid (PFBS) 1.9 mg/kg, dry weight (1,900 ng/g)
- Perfluorooctane sulfonate (PFOS) 0.0052 mg/kg, dry weight (5.2 ng/g)
- Perfluorooctanoic acid (PFOA) 0.0025 mg/kg, dry weight (2.5 ng/g).
Canada
In 2017 Environment and Climate Change Canada published Federal Environmental Quality Guidelines for PFOS. This document limits PFOS in soils and sets this limit at 0.01 mg/kg (10 ng/g) for agricultural soils. The same limit is also applied to residential/parkland soils, with a higher limit for commercial and industrial soils.
PFOS, PFOA and compounds that consist of specific perfluorinated alkyl groups were included in the 2012 Canadian Prohibition of Certain Toxic Substances Regulations, which limited their use to photolithography, printing and aqueous film forming foams.
China
As of March 2020, there have been a total of six studies into PFAS contamination of biosolids from Chinese wastewater treatment plants and one study for Hong Kong. The maximum concentration reported was for PFOS found in primary sludge in Hong Kong (7,304 ng/g); for mainland China the maximum reported mean concentration for PFOS was 5,383 ng/g (Guo et al., 2008). As there have been studies that investigated PFAS contamination of wastewater sludge, it can be inferred that China is aware of PFAS contamination in domestic and industrial wastewater.
A search of the internet for Chinese regulations regarding PFAS loads in biosolids and sludges did not reveal any results, and this may imply that there is currently no guidance or regulation governing PFAS loads in biosolids or sludge. According to the OECD (2015), as of 2008, the Chinese Ministry for Environmental Protection (MEP) included PFOA as a part of their “High Pollution, High Environmental Risk Product Catalogue.” The OECD also reported that in 2011 the National Development and Reform Commission issued “Industrial Reconstructuring Guide Directory”, which placed restrictions on PFOS and PFOA. Following this, in 2014, the MEP issued announcement No. 21, which banned the importation, transport, manufacture, production and export of PFOS and PSOSF.
A particularly interesting investigation by Zhang et al. (2013) found a strong positive correlation between the concentration of PFCAs, PFOS and PFHpA in wastewater and the gross domestic product of cities in China.
Singapore
As of September 2019, PFOA and its salts have been added to a control list by Singapore’s National Environmental Agency (NEA). A search of the internet has not revealed any documents regarding PFAS regulations in biosolids. To date there has only been one published Singaporean study of PFAS in biosolids. PFOS concentrations ranged between 2.9 ng/g (influent particulate) to 702.2 ng/g (digested sludge) (Yu et al., 2009).
South Korea
Only as recently as 2005 has South Korea banned biosolids and sludge from being dumped in landfills or disposed of in the ocean, which in turn has led to a focus on investigating potential beneficial reuse of the resource (Ahn and Choi, 2004). A search of the internet revealed no publicly available documents that detail PFAS regulations concerning wastewater sludge or biosolids in South Korea. PFOS and its salts are listed as restricted substances under South Korea’s Persistent Organic Pollutants Control Act, except for exemptions specifically detailed in the Stockholm Convention. South Korea has been conducting ongoing environmental monitoring for PFOS since 2013, and 2015 for PFOA.
The first South Korean study to investigate PFAS in biosolids was a 2010 published study by Guo et al. (2010), which analysed the sludge of 20 South Korean WWTPs. PFOS was detected in 100% of municipal sludge samples. The highest PFOS mean reported was 54.1 ng/g. Following this, another South Korean study by Kim et al (2012) found the maximum PFOS level was 1,200 ng/g (mean 110 ng/g).
Japan
Beneficial reuse of biosolids in Japan has been gradually increasing since the 1990s up to 60% in recent years (UN-Habitat, 2008). Japan does appear to have an incineration policy for disposing of solid wastes contaminated with PFAS, but there appears to be no publicly available policy document detailing PFAS limits in Japanese biosolids. As a signatory to Annex B of the Stockholm Convention, Japan has a Class 1 export restriction for PFOS and its salts. Japan has been conducting environmental testing for PFOS since 2009 (OECD, 2015).
There has been one published study that confirms PFAS in Japanese wastewater and sludge (Shivakoti et al., 2010), however the PFAS concentration detected in sludge was quantified as a liquid, making it impossible to compare the PFAS concentration to those quantified as dry weight solids. Japan is the third largest manufacturer of paper products in the world and as paper manufacturing is commonly recognised as a direct source of PFAS contamination to wastewater, it is highly likely that Japan has a large amount of PFAS contamination in their biosolids.
India
India currently has no regulations regarding PFAS, although it has been detected in surface waters, groundwater and biota. As the volume of sewage treatment in India is minimal when compared to western standards, this will change as India connects more villages to centralised wastewater treatment and sludge disposal becomes an issue. As India has a very large manufacturing sector, including textiles processing and electronics, it is likely that there is widespread environmental PFAS contamination. However, this is expected to be caused by direct contact with untreated (or minimally treated) manufacturing wastewater, rather than via biosolids.
There have been no published studies on PFAS concentrations in Indian biosolids.
Central and South America
Currently there are no published regulations on PFAS contamination for South American biosolids. An internet search revealed no regulations regarding PFAS in any South American country. Both Argentina and Brazil are signatories to the Stockholm Convention and have ratified the amendments to Annex B, which restricts PFOS use.
There has been no study of PFAS contamination of wastewater in Argentina and Brazil. However, there has been an acknowledgement that the manufacture and use of the pesticide Sufuramid to control leaf cutter ants in plantation eucalypts is a source of environmental PFOS contamination (Nascimento et al., 2018). Sulfuramid usage is widespread in Uruguay, Brazil, Argentina, Paraguay, Colombia and Venezuela. Under Annex B of the Stockholm Convention, PFOS restrictions are exempt for uses such as “Insect baits for control of leaf-cutting ants.”
PFAS has been detected in Brazilian drinking water samples (Schwanz et al., 2016) which gives a strong indication that it may also be present in Brazilian biosolids. PFAS has also been detected in the ground and surface waters of French Guiana, Martinique and Guadeloupe (Munoz et al., 2017).
As wastewater infrastructure increases in South America, the production of biosolids will also increase and as such, so will the need to find beneficial uses for the newly acquired, and quickly accumulating, biosolids. Continued development, along with the use and manufacture of PFAS-containing pesticides, will likely result in an increasing PFAS concentrations in biosolids.
Africa and developing countries
The UN-Habitat Global Atlas of Excreta 2008 states that many African countries lacks basic sanitation facilities for large sections of the population. With little to no wastewater infrastructure, the main focus is to implement sanitation infrastructure to improve human health and reduce pollution. Therefore, it is not expected that any African countries would have yet considered regulating PFAS in biosolids.
According to the United Nations World Water Development Report 2017, 2.4 billion people did not have access to improved sanitation and nearly one billion people worldwide were practising open defecation. This report also acknowledged that in some parts of the developed world, informal reuse of untreated wastewater was occurring without any appropriate safety measures or oversight. The report also states that, as of 2015, 92% of wastewater in developing low-income countries is not treated. This provides some perspective of the gulf in biosolids management between developing and developed countries.
To date there have been two studies that have investigated PFAS contamination of municipal and industrial produced sludge and biosolids including Nigeria (Sindiku et al., 2013) and Kenya (Chirikona et al., 2015). Both of these studies reported concentrations in picogram per gram (pg/g) range, and as such, the reported PFAS concentrations were orders of magnitude below those reported in Asia, Europe and the USA.
Relevance for Australia
PFAS in biosolids presents an incredibly challenging problem in developed countries because of community concern and limited treatment options. It will be present in virtually all biosolids in Australia, however, cannot readily be treated without destroying the entire biosolids volume. The answer to this problem cannot be found overseas; few countries have implemented action and there is no consensus on the approach to setting limits for use. Therefore, how can we continue to safely apply biosolids to land? Until there is consensus on a threshold limit based on rigorous science, perhaps a more nuanced solution is warranted. Several approaches are discussed, as follows.
Source control is a proven strategy for managing contaminants. A study of the biosolids from 1165 German wastewater treatment plants by Ulrich et al. (2016) demonstrated that the total load of perfluoroalkyl substances decreased by more than 90%. This decrease was measured between 2008 and 2013, when the European Union prohibited the general use of PFOS and major manufacturers voluntarily phased out PFOA use.
Australia currently has limited published data on PFAS in raw wastewater. We expect individual utilities are testing for PFAS in their wastewater streams, but this data is fragmented. There is a need to bring this information together to understand how source control could be used to reduce PFAS in biosolids and recycled water. Ongoing testing and research would then be needed to demonstrate the effectiveness of regulation and source control measures.
There also appears to be a lack of comparative Australian data, for example, regarding metropolitan versus regional catchments. It is very likely that the concentration of PFAS in biosolids will be related to the number of trade waste customers and the presence of contaminated groundwater in any given catchment. By sharing data on PFAS concentrations in their biosolids, the industry as a whole would be better informed of where the risks lay. That is, targeted management of the highest risk biosolids could be implemented by reducing environmental impact and allowing lower risk biosolids to be beneficially used.
We should also acknowledge that a simple limit for PFAS in biosolids may not be an achievable goal. While this is neat and convenient, and has been the approach to historic contaminants such as heavy metals, it does not reflect the complex matrix of chemicals and interactions that occur in biosolids and receiving environments. We may never be clearly able to define what a safe concentration is because there are too many variables. Therefore, taking a risk-based approach by prioritising management of the most contaminated biosolids could lead to the most practicable outcome.
Summary
PFAS contamination in the environment is strongly linked to the level of development and industrialisation in any given country. This is reflected in the international data on the concentration of PFAS in biosolids, despite the limited data. The main contributing factors to European regulation of PFAS in soils seem to be historical incidents of contamination, the fate of biosolids, and the level of development. This is also true for the USA, Canada and Australia.
For many of the world’s developing regions and nations, while PFAS may be present in wastewater biosolids at trace amounts, it is currently not cause for regulation when compared to the issues relating to a lack of sanitation. This includes safe and sustainable biosolids disposal pathways.
As increased PFAS concentrations in wastewater have been correlated with increased GDP, it is likely that as economic growth continues, so too will the concentrations of PFAS in biosolids. The development of strategies to control point and diffuse PFAS sources before they reach wastewater treatment plants would be an ideal scenario. This would also be dependent, in part, on long-term political commitments to adhere to the Stockholm Convention annexes that regulate PFAS.
Germany and Maine, USA, have set limits for PFAS in biosolids for land application, and we believe these are the only locations in the world to have done so. Although some countries and states have implemented limits for PFAS in soils, there does not appear to be any consistency in the approach, nor the upper limits.
Both Norway and Finland are using national risk-based approaches, rather than setting specific limits. The Australian water industry could implement a similar approach, acting collectively to identify high-risk biosolids and implement management actions to target these biosolids. This approach does not prevent limits from being implemented in the future, but it could provide a pathway to addressing the environmental risks of PFAS in biosolids.
About the authors
Hilary Hall | Hilary is a process engineer and Senior Consultant at RMCG, with expertise in the treatment and management of wastewater, trade waste, biosolids and recycled water. Hilary has particular experience in wastewater nutrients and contaminants; how they are affected by treatment processes and how they impact beneficial use.
Damien Moodie | Damien is a PhD candidate at RMIT University, researching contaminants in biosolids since 2015. His work includes the study of microplastics, PFAS and polybrominated chemical in biosolids. Damien has an excellent understanding of contaminant concentrations in both international and Australian biosolids.
Catherine Vero | Catherine is a Principal Consultant with PSD, specialising in biosolids management. Cath has extensive experience in biosolids management throughout Australia and has a particular interest in developing practical solutions to complex biosolids management problems.
References
Ahn, Y-H and Choi, H-C (2004) Municipal sludge management and disposal in South Korea: Status and a new sustainable approach. Water Science and Technology, 50, 245-253.
Chirikona, F, Filipovic, M, Ooko, S and Orata, F (2015) Perfluoroalkyl acids in selected wastewater treatment plants and their discharge load within the Lake Victoria basin in Kenya. Environ Monit Assess, 187, 238.
Council of Australian Governments (2020) Intergovernmental Agreement on a National Framework for Responding to PFAS Contamination.
Danish EPA (2018) Risk assessment of fluorinated substances in cosmetic products.
Danish EPA (2015) Perfluoroalkylated substances: PFOA, PFOS and PFOSA. Evaluation of health hazards and proposal of a health based quality criteria for sinking water, soil and ground water, Environmental project No. 1665, 2015.
D’Eon, J, Crozier, PW, Furdui, VI, Reiner, EJ, Libelo, EL and Mabury, SA (2009) Observation of a commercial fluorinated material, the polyfluoroalkyl phosphoric acid diesters, in human sera, wastewater treatment plant sludge, and paper fibres. Environ. Sci. Technol. 43, 4589-4594.
De Silva, A O, Allard, C N, Spencer, C, Webster, G M and Shoebi, M (2012) Phosphorus-containing fluorinated organics: polyfluoroalkyl phosphoric acid diesters (diPAPs), perfluorophosphonates (PFPAs), and perfluorophosphinates (PFPIAs) in residential indoor dust. Environ Sci Technol, 46, 12575-82.
Environment Protection Authority Victoria (2020) Interim position statement on PFAS, Publication 1669.4.
Fujii, Y, Harada, K H and Koizumi, A (2013) Occurrence of perfluorinated carboxylic acids (PFCAs) in personal care products and compounding agents. Chemosphere, 93, 538-44.
Gallen, C, Drage, D, Kaserzon, S, Baduel, C, Gallen, M, Banks, A, Broomhall, S & Mueller, JF (2016) Occurrence and distribution of brominated flame retardants and perfluoroalkyl substances in Australian landfill leachate and biosolids, J Hazard Mater, vol. 312, pp. 55-64.
German Federal Environment Agency (2009), Do without perfluorinated chemicals and prevent their discharge into the environment.
Guo, R, Sim, W-J, Lee E-S, Lee, J-H and Oh, J-E (2010) Evaluation of the fate of perfluoroalkyl compounds in wastewater treatment plants. Water Research 44, 3476-3486.
Guo, R, Zhou, Q, Cai, Y and Jiang, G (2008) Determination of perfluorooctanesulfonate and perfluorooctanoic acid in sewage sludge samples using liquid chromatography/quadrupole time-of-flight mass spectrometry. Talanta, 75, 1394-9.
Kim, S K, Im, J K, Kang, Y M, Jung, S Y, Kho, Y L and Zoh, K D (2012) Wastewater treatment plants (WWTPs)-derived national discharge loads of perfluorinated compounds (PFCs). J Hazard Mater, 201-202, 82-91.
Kotthoff, M, Muller, J, Jurling, H, Schlummer, M and Fielder, D (2015) Perfluoroalkyl and polyfluoroalkyl substances in consumer products. Environ Sci Pollut Res Int, 22, 14546-59.
Munoz, G, Labadie, P, Botta, F, Lestremau, F, Lopez, B, Geneste, E, Pardon, P, Devier, M H and Budzinski, H (2017) Occurrence survey and spatial distribution of perfluoroalkyl and polyfluoroalkyl surfactants in groundwater, surface water, and sediments from tropical environments. Sci Total Environ, 607-608, 243-252.
Nascimento, R A, Nunoo, D B O, Bizkarguenaga, E, Schultz, L, Zabaleta, I, Benskin, J P, Spano, S and Leonel, J (2018) Sulfluramid use in Brazilian agriculture: A source of per- and polyfluoroalkyl substances (PFASs) to the environment. Environ Pollut, 242, 1436-1443.
NICOLE (Network for Industrially Contaminated Land in Europe) (2016), Environmental fate and effects of poly- and perfluoroalkyl substances (PFAS), report no. 8/16.
OECD (2015) Risk Reduction Approaches for PFASs – A Cross-Country Analysis, OECD Environment, Health and Safety Publications Series on Risk Management No, 29.
OECD (2018) Towards a new comprehensive global database of per- and polyfluoroalkyl substances (PFASs): Summary report on updating the OECD 2007 list of per- and polyfluoroalkyl substances (PFASs)
PSD (2019) ANZBP Biosolids Production in Australia.
Queensland Government (2019) End of Waste Code Biosolids (EMEW07359617).
RIVM (National Institute for Public Health and the Environment, The Netherlands) (2020) Newsletter 2020, issue 1.
Sahlin,S, for Swedish EPA (Environmental Protection Agency) (2017) PFAS in the Baltic Sea Region.
Schwanz, T G, Llorca, M, Farre, M and Barcelo, D (2016) Perfluoroalkyl substances assessment in drinking waters from Brazil, France and Spain. Sci Total Environ, 539, 143-152.
Shivakoti, B R, Tanaka, S, Fujii, S, Kunecheva, C, Boontanon, S K, Musirat, C, Seneviratne, S T and Tanaka, H (2010) Occurrences and behavior of perfluorinated compounds (PFCs) in several wastewater treatment plants (WWTPs) in Japan and Thailand. J Environ Monit, 12, 1255-64.
Sindiku, O, Orata, F, Weber, R and Osobanjo, O (2013) Per- and polyfluoroalkyl substances in selected sewage sludge in Nigeria. Chemosphere, 92, 329-35.
Stahl, T, Gassmann, M, Falk, S and Brunn, H (2018) Concentrations and distribution patterns of perfluoroalkyl acids in sewage sludge and in biowaste in Hesse, Germany. J. Agric. Food Chem. 66, 10147 – 10153
Trier, X, Granby, K and Christensen, J H (2011) Polyfluorinated surfactants (PFS) in paper and board coatings for food packaging. Environ Sci Pollut Res Int, 18, 1108-20.
Ulrich, H, Freier, K P and Gierig, M (2016) Getting on with persistent pollutants: Decreasing trends of perfluoroalkyl acids (PFAAs) in sewerage sludge. Chemosphere, 161, 527-535.
UN-Habitat (2008) Global atlas of excrete, wastewater sludge, and biosolids management: moving forward the sustainable and welcome uses of a global resource.
Venkatesan, AK & Halden, RU (2013) ‘National inventory of perfluoroalkyl substances in archived U.S. biosolids from the 2001 EPA National Sewage Sludge Survey’, J Hazard Mater, vol. 252-253, pp. 413-8.
Yu, J, Hu, J, Tanaka, S and Fujii, S (2009) Perfluorooctane sulfonate (PFOS) and perfluorooctanoic acid (PFOA) in sewage treatment plants. Water Res, 43, 2399-408.
Zaferiaki, E, Costopoulou, D, Vassiliadou, I, Bakeas, E and Leondiadis, L (2014) Determination of perfluorinated compounds (PFCs) in various foodstuff packaging materials used in the Greek market. Chemosphere, 94, 169-76.
Zhang, W, Zhang, Y, Taniyasu, S, Yeung, L W, Lam, P K, Wang, J, Li, X, Yamashita, N and Dai, J (2013) Distribution and fate of perfluoroalkyl substances in municipal wastewater treatment plants in economically developed areas of China. Environ Pollut, 176, 10-7.