How much water supply resilience is enough? – A discussion paper
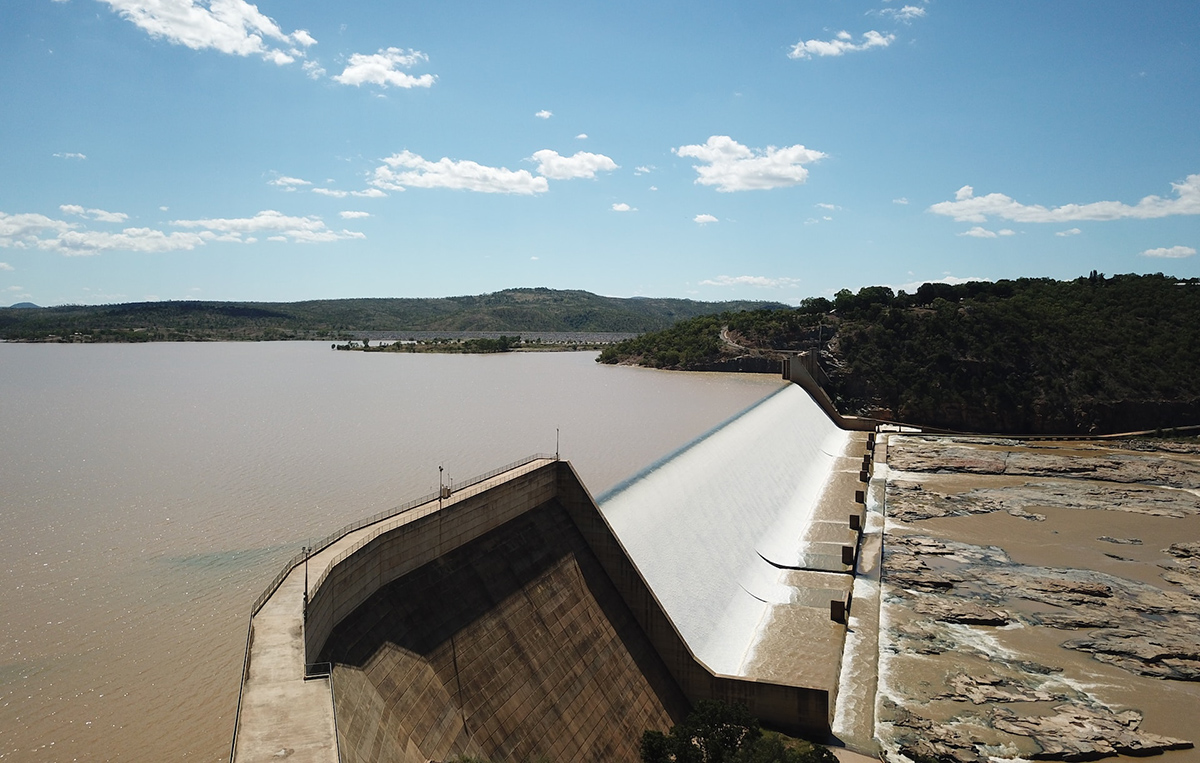
DOWNLOAD THE PAPER
Abstract
Resilience for water supply systems is the ability to maintain supply when the system is impacted by a disruption from the norm. It is not just about source reliability. It includes all manner of infrastructure failures and natural disasters, and even terrorist and war events. There is a spectrum of failure scenarios ranging from relatively short local supply area outages through to city wide total losses of supply, and the associated consequences range from inconvenience to catastrophic. Whatever the nature of a supply disruption, there will be a resilience measure which could be taken to reduce its impact, but could it be justified particularly when there is a small probability of it ever being needed. It is proposed guidance about how much resilience is needed could be obtained from existing level of service standards, even though those standards might not be used locally or be directly related to water supply.
The paper investigated two ends of the resilience spectrum, frequently and exceptionally infrequently resilience needed events. It was found that most Australian water supply authorities are providing a similar level of service, and consistent with a 10 to 15-year standard for frequently occurring resilience events. Storage tanks are the main source of resilience for many water supply areas for such events and have historically been sized using qualitative methods. This paper discusses the more effective use of quantitative methods. At the other end of the spectrum resilience is capable of maintaining supply in events with average recurrence intervals of 1,000 to <10,000 years is specified by published emergency risk guidelines. A high-level review of Queensland urban centres found resilience to meet all types of failure scenarios, not just droughts, approaching a 10,000-year standard could be provided in conjunction with high level of water restrictions if independent water source and treatment capabilities from adjoining supply areas were interconnected.
Introduction
Resilience, for water supply systems, is defined in this paper as the ability to maintain supply when the system is impacted by a disruption from the norm. The range of possible water supply disruptions is large and can include mechanical and infrastructure failures, power supply outages, droughts, floods, earthquakes and even terrorist and war actions.
Resilience measures can take many forms. For example, drought resilience can often be improved by increasing dam storages, mechanical failure resilience can be improved by providing standby/duty arrangements, and power outage resilience can be improved by increasing the volume of emergency storage in a distribution system’s tanks. Notably, resilience measures can be enhanced by developing interconnections with other supply areas with independent water supplies.
There are many drivers which can change the extent of water supply resilience measures. These might include increases in demand caused by population growth, per capita consumption growth or poor management of system losses. Reductions in source water supplies associated with climate change is another driver often raised as a resilience concern.
This paper does not discuss resilience drivers; its intent is to identify existing Australian standards which could be adopted to establish how much water supply resilience is enough, and to discuss strategies for providing that resilience.
Level of service approach
Whatever the nature of a supply disruption, there will be a resilience measure which could be taken to reduce its impact. Of course, some resilience measures might seem too costly, and the question will arise about their justification, particularly when there appears to be a small probability of them ever being needed. Such questions can sometimes be resolved using economic assessment methods where the community is asked how much they would be prepared to pay to avoid supply disruptions. These methods rely on community survey responses and are most valid where emergency events are expected to occur relatively frequently, say, more often than once in 50 years. However, the economic costs of disruptions, i.e., emergencies, become insignificant in present day terms as their frequencies of occurrence reduce to include rare events. The use of economic assessments for evaluating the resilience requirements of rare events is therefore not useful.
While water supply resilience requirements can be reduced by applying non-engineering strategies such as demand management, resilience provision is generally an engineering problem. Historically, setting standards for these types of engineering problems has been tackled by developing local “rules” about what is an acceptable level of service. Over time those rules have been compared among like communities and standards have been revised to conform with what had been learnt and applied elsewhere.
For example, water restrictions which were only operated in a few parts of Australia 100 years ago have now been adopted by most Australian water authorities. The levels of service of those restrictions have progressively become uniform to the point that most Australian cities have now chosen frequencies of between 10 and 20 years average recurrence interval for their first level or stage of restrictions (Killen, 2019). It has been suggested that this process of standards becoming more uniform is driven by peer comparisons being made between water authorities.
Resilience spectrum
The term resilience does not mean the same for all loss of water supply situations. Indeed, there is a spectrum of resilience situations and consequences.
At one end of the spectrum is a distribution area serving, say, a hundred residential properties which is dependent on the storage volume within its local tank to maintain supply if there is a system failure, such as a break of the pipe feeding that tank. Resilience here would be defined as the capacity of the tank to maintain supply for the duration of the pipe repair, perhaps only a few hours. The consequences of not having sufficient storage would be primarily one of inconvenience associated with the brief loss of supply for the area’s consumers, albeit with possibly some embarrassment to the local supply authority.
At the other end of the spectrum is an extended loss of supply to a city with, say, a million residents. That loss of supply might be because, say, an earthquake had damaged the city’s only water treatment plant, with perhaps twelve weeks before it could be returned to operation.
Resilience in this situation would be defined as the capacity to maintain supply, though possibly heavily restricted, for the twelve-week treatment plant repair period. The consequences of not being able to maintain a water supply for that period could be catastrophic for many communities.
The discussions which follow focus on these two ends of the resilience provision spectrum.
Frequently needed resilience
Nature of frequent resilience events
Events which frequently need resilience support are of short durations, i.e., less than a few days. They are usually related to infrastructure failures such as pipes, pumps and power supplies but can also include raw water quality exceedances, e.g., high turbidity and cyanobacteria. Notably, peaks in demand such as the maximum day demand are also part of this resilience events set.
The consequences of these events, if the system had insufficient resilience, would generally be limited to community inconvenience, possibly associated with political embarrassment.
Relevant level of service standards
Urban water supply systems are commonly developed to supply peak day demands with losses of supply infrequently occurring due to insufficient hydraulic capacity or infrastructure failures. But the definition of “infrequently”, i.e., what is an acceptable level of service for the reliability of water supply systems is not well documented.
The Hunter Water version of the Water Supply Code of Australia (WSAA, 2009) provides both peak day and extreme day demand peaking factors. The extreme day demand peaking factors are expected to occur about every 10 to 15 years on average and are used in the Hunter Water code for determining both continuity of supply and tank storage requirements.
That 10 to 15-year level of service standard is generally supported by an analysis of Australian water supply authority water interruption rates for the 10-year period 2011 to 2021 as reported in the National Performance Report 2020–21: Urban Water Utilities (BOM, 2022). The analysis found an average rate of supply interruptions of 12.6 years, and the annual interruption rate ranged from 10.8 to 19.9 years. That water interruption data was collated from 87 supply authorities with property connection numbers ranging from 10,000 to over 100,000.
Distribution tanks as a resilience measure
The role of a tank in a water distribution area is to balance out peaks in demand against the available inflow, and to provide a reserve supply to meet demand when supply to the area is reduced or stopped. Tanks are the only source of resilience for many water supply areas without connection to another area.
Storage tanks in Australia are commonly sized using the Water Supply Code of Australia (WSA-03, 2011). That code advises that resilience capabilities of tanks should be based on Risk Management standard, AS/NZS 4360. That standard uses a qualitative methodology which is conservative by nature. This paper proposes a quantitative methodology which has been successfully used by several South East Queensland water authorities for considering their storage tanks’ resilience roles. (A Queensland Urban Utilities paper presented at Ozwater ’23 (Wilson, 2023) discussed the advantages of using that methodology).
The methodology takes guidance from Australian Standard, AS 2419.1, Fire hydrant installations System design, installation and commissioning. Calculation of the storage for a fire event is documented as that needed to meet fire-fighting demands in conjunction with the 95th percentile supply area demand. Commonly, two-thirds of the maximum day demand is used to represent the 95th percentile value.
This concept of the demand associated with an “event” being less than the maximum day demand is discussed in the AWA e-Journal paper, Water Supply Risk Assessments Using Stochastic Peaking Factors, (Donaldson, 2018-1) and a mathematical relationship between the demand peaking factor, the probability of the “event’s” occurrence and the supply area’s level of service is developed. The three components of that mathematical relationship are:
- The probability of a failure event, e.g., a pump power outage or a pipeline break for the inflow to a supply area tank can be estimated using Boolean algebra as discussed in the AWA e-Journal paper, Sizing Water Distribution Storages for Persistence and Emergency Demands, (Donaldson, 2018-2).
- The level of service for the supply area.
- The resultant demand associated with the “event” is a demand percentile peaking factor in the same manner that the 95th percentile has been adopted for fire events. Many annual daily demand data sets have been analysed for South East Queensland to provide stochastic peaking factors for the 100th, 99th, 98th, 95th, 90th, 80th, 70th, 60th and 50th demand percentiles. Similar peaking factors can be prepared for any supply area where a ten-year record, or greater, of daily demand records is available for analysis.
A Microsoft Excel based tool (Tank Sizing Tool) has been developed which lets the user analyse a distribution system’s tank demands and its inflow infrastructure reliability. A plot of inflow failure rates versus outage times is generated and the maximum storage required to maintain supply is calculated for the range of possible failure events within the required level of service, i.e., frequency of supply failure. The tool also calculates how much storage is required for non-failure events, and the overall maximum storage required to meet all inflow failure and non-failure demand events.
A link to this tool, pre-loaded with South East Queensland stochastic peaking factors, can be found here. The link includes a simple example of a typical pumped pipeline supplying a storage tank plus guidelines for use of the tool. The Tank Sizing Tool uses a supply area’s level of service as its resilience criteria. The resilience of any supply area which relies on a single tank can be determined using this tool.
Determination of the resilience of distributions systems which interlink reservoirs with multiple inflows sometimes requires more powerful tools, but the same mathematical relations between probability of failure, probability of demand and the required level of service apply. The South East Queensland water authority, Seqwater, operates a complex water grid including 15 water treatment plants supplying over 160 tanks. It uses the Resilience Model which allows the determination of the minimum storage requirements of both individual tanks and groups of tanks for both maximum demand and system failure scenarios. That model utilises non-linear equation software to also solve resilience questions such as the minimum required tank storage volumes, or minimum water production requirements of individual water treatment plants, groups of water treatment plants, etc.
Summary
The Hunter Water version of the Water Supply Code of Australia used for determining both continuity of supply and tank storage uses extreme day demand peaking factors which are expected to occur about every 10 to 15 years on average. Interruption rates collated by BOM indicate that Australian water supply systems are generally operating at a similar level of service.
Most water supply systems are frequently impacted by maximum day demand, fire flow and reduced/stopped inflow “emergency” events. Resilience to maintain water supply during these events is commonly provided by tank storage. Tools such as the Tank Sizing Tool and the Resilience Model can be used to investigate the resilience of any water supply system with respect to the level of service adopted by its water supply authority.
Exceptionally infrequent needed resilience
Nature of infrequent resilience events
Exceptionally infrequent resilience events have long durations extending for weeks or even months. They are generally caused by natural events such as extreme floods, cyclones and earthquakes but might also result from failures in critical infrastructure where repairs are difficult or slow to execute. They can also include natural disasters and terrorist and war actions.
Their frequency is commonly in the order of 1 in 1,000 plus years and as such probabilities of otherwise usually discounted failure events need to be considered. The consequences of such events for many communities could be catastrophic. At best there would be a need for massive community relocation in association with economic breakdown. At worst there could be large losses of life because humans can generally only survive for about three days without water.
Relevant level of service standards
Like frequently required resilience, there are few existing Australian level of service standards for infrequent resilience required events. However, standards which have been developed in recent years for severe drought restrictions do provide some guidance. Table 1, adapted from Killen, 2019, lists infrequent event, i.e., 1,000 year and greater, standards (in years) taken up by some Australian cities.
Table 1: Severe drought restrictions for Australian Cities
The Table 1 South East Queensland restrictions are unique in Australia in their being made into a legislated regulation.
The purpose of that regulation is to define the minimum supply, named the Essential Minimum Supply Volume (EMSV), that the area’s water sources need to be able to provide in an extended drought. The concept is that a supply area’s basic water needs will always be met, i.e., there would never be a situation where a community could perish because of loss of its water supply. Basic water needs include water essential for drinking and hygiene and for essential services such as hospitals, adequate food production and associated power generation.
The regulation requires that there should be enough water to provide the essential minimum supply at an average of 100 litres per person per day (Lpd), excluding system losses, and that such shortages of supply will not occur more often than once in 10,000 years, on average. The regulation also ensures that there is enough water maintained in each of the key sub-regions to ensure continuity of supply in the event of breakdowns in water transfer arrangements.
While some other Australian water supply authorities are required to operate their dams so that they only infrequently reach set minimum levels, the South East Queensland regulation is the only known formal use of a minimum supply requirement as a resilience mechanism.
Use of essential minimum supply volume resilience for other emergencies
Would the EMSV concept for drought resilience also be appropriate for infrastructure failure related resilience? In that regard, the two main criteria of the drought related EMSV legislation, the 10,000-year frequency and the 100 Lpd minimum supply rate, are discussed further:
10,000-year frequency
The 10,000-year frequency number was adopted to include the occurrence of an extreme drought event and was primarily meant to act as a buffer against possible economic ruin. The South East Queensland bulk water supply services around 70% of the population of Queensland and therefore a SEQ bulk water supply failure would have enormous economic impacts for the State. (DNRME, 2019) Seventy percent of the Queensland population is about 2.1 million people. A lesser frequency, down to 1,000 years, would be more appropriate for smaller supply areas. That would be consistent with the likelihood of occurrence of very rare events shown highlighted in Table 2, reproduced from the National Emergency Risk Assessment Guidelines (NERAG, 2015).
Table 2: National Emergency Risk Assessment Guidelines Likelihood Table
Droughts take years for their full impacts to be realised whereas infrastructure failures occur with little warning, and time to make contingency provisions. Some infrastructure failures can impact the whole supply system and cause outages with durations of weeks or even months. These failures typically would impact a supply area’s source water and treatment infrastructure and would be associated with natural events such as floods, bushfires, and earthquakes.
Other possible infrastructure failure causes listed in the Queensland Emergency Risk Management Framework (QERMF, 2015) include:
- An explosion or fire, a chemical, fuel or oil spill, or a gas leak;
- A failure of, or disruption to, an essential service or infrastructure; and
- An attack against the State.
The consequences of a critical infrastructure failure with an extended loss of water supply could be catastrophic for many communities. The survival of community members would be dependent on household rainwater tank storage volumes at the time of the event, and emergency measures such as bottled water distributions.
While small communities of less than, say, 10,000 people could be maintained by trucked water from other areas, larger communities would have to be evacuated to survive. Such catastrophic events should occur very to extremely rarely and Table 2 indicates that an average recurrence interval of 10,000 to > 10,000 years would be applicable. But if an EMSV could be maintained, a possible catastrophe could be avoided and a 1,000 to 10,000 average recurrence interval would become applicable, i.e., the same resilience standards discussed above for a drought depleted water supply in association with an EMSV would also apply.
100 Lpd minimum supply rate
Bross et al, 2019, reported that while there are United Nations emergency supply guidelines for drinking water supplies, few countries with reticulated systems have minimum water supply guidelines for disaster events. Germany was quoted as a country which requires a minimum of 50 Lcd supply to be maintained from its reticulated systems with additional per bed supplies for hospitals. The South East Queensland 100 Lcd standard appears to draw from the German guidelines:
International research indicates that the minimum residential use to sustain life is approximately 50 litres per person per day for residential water use. Recent SEQ regional estimates for minimum non-residential use range from 15–20 litres per person per day (based on actual water use from January to March 2013). The lower end of the estimate includes water use for hospitals, power stations and particular industries that must continue to operate to ensure public safety. The higher end of the estimate includes water use for other heavy industries, oil refineries, and hotels/motels (DNRME, July 2019).
It is noted that the provision of EMSV to some supply areas outside of South East Queensland might not need to provide water for power stations and industries and the 100 Lcd allowance might be reduced to suit.
Use of redundancy for achieving high levels of resilience
Resilience improvement measures can generally be divided into those which improve reliability, and those which provide redundancy. Generally, improvements based on providing redundancy will more effectively increase a system’s resilience than making infrastructure improvements. For example, an infrastructure item with a failure probability of, say, 1 in 50 years might be readily upgradable to a 1 in 200-year failure probability by replacing some aged components. But providing a parallel redundant item of the same reliability standard would, using simple Boolean algebra, increase the overall failure probability, i.e., its resilience, to 50 x 50 = 2,500 years. This is clearly a much better resilience than the 1 in 200 years achieved by solely upgrading the existing item’s reliability.
Redundancy of water supply systems can be created by interconnecting supply areas, and their independent source and water treatment capabilities. This concept is well known as a resilience improvement measure and is discussed as a basic resilience option in Planning for an Emergency Drinking Water Supply, (US EPA, 2011). Inclusion of the EMSV concept encourages the interconnection of water supplies because it enables small capacity systems to be utilised to support adjacent areas so long as the overall EMSV can be provided.
Summary
Exceptionally infrequent resilience events can include all manner of emergencies, e.g., explosions, infrastructure failures, earthquakes, fires, floods, terrorist acts and wars, and are not limited to drought events.
The consequences of an occurrence of such an event for many communities could be catastrophic. Emergency risk guidelines such as The National Emergency Risk Assessment Guidelines and the Queensland Emergency Risk Management Framework provide guidance for the level of service required for the continuation of water supplies in the event of such resilience events.
A level of service between 1,000 and 10,000 years is indicated. That is consistent with the EMSV standard required in South East Queensland for drought resilience purposes, by regulation. Such high levels of service can most effectively be achieved by providing interconnections between two adjacent supply areas with independent water sources and treatment facilities so long as the smaller supply capacity is sufficient to meet the combined area’s EMSV.
Discussion
Resilience at ends of resilience spectrum
It was found that most Australian water supply authorities are providing similar levels of service for frequently occurring resilience related events, and to standards which are consistent with the only identified Australian standard, the Hunter Water version of the Water Supply Code of Australia. That resilience is primarily provided by storage held in distribution tanks. That code recommends the use of qualitative methods for assessing tank storage resilience requirements. This paper proposes the use of more definitive quantitative methods which have been effectively used by some South East Queensland water authorities.
Supply resilience for extremely infrequently occurring events has often been considered and provisions made by many authorities with respect to drought impacts. However, resilience requirements for natural events such as floods, bushfires, and earthquakes, infrastructure failures and attacks against the State, have generally only been seen as a collateral benefit after meeting drought requirements. The National Emergency Risk Assessment Guidelines indicate that resilience capable of maintaining supply in events with average recurrence intervals of 1,000 to <10,000 years should be provided. That is consistent with the EMSV concept legislated for South East Queensland which requires a 1 in 10,000-year level of service for drought resilience in conjunction with a high level of water restriction.
The effectiveness of achieving high levels of service by providing interconnections between two adjacent supply areas with independent water sources and treatment facilities was identified. But could that concept work in practice? Are there many supply areas which could reasonably access two independent source and supply systems? A high-level assessment of Queensland’s south east water grid and regional water supply areas was undertaken to investigate these questions. (It was assumed in the assessments that communities with populations less than 10,000 persons could, in an emergency, be supplied by water trucked from other areas). It was found that there are 25 urban water supply schemes in Queensland with populations greater than 10,000. Of those 25 schemes, 17 now have two, or more, independent source and treatment systems which would allow for one supply to fail while maintaining an EMSV. (Many of the 17 existing schemes are part of the South East Queensland water grid).
It was also found that new source and water treatment plants, and the construction of new inter-scheme pipelines are in progress for three additional schemes. It is most notable that all the schemes able to maintain an EMSV were primarily developed to meet drought resilience water security objectives. In practice, while some of the schemes have since been used for water security purposes, all been used much more for other resilience purposes, in particular maintaining supply during infrastructure failures.
It is likely that the reason for basing infrequent resilience schemes on drought events is because droughts occur relatively often and have a large range of durations and intensities, most of which will have some impact on their supply systems. Most of the other types of resilience events, e.g., infrastructure failures generally have short durations and can be coped with by tank storages, whereas the events which could cause catastrophic losses of supply have very low frequencies of occurrence.
The development of interconnected independent water supply schemes will provide the best resilience outcomes, but it could sometimes be difficult to put forward a successful business case for the associated infrastructure works based on resolving risks which are not drought related, and which have low probabilities of occurrence. It is suggested that a strategy for developing a scheme capable of providing all forms of resilience could be to leverage off drought resilience proposals and argue, say, if there a choice between raising an existing dam and interconnecting with another supply scheme, that the latter should be chosen because of the broader range of resultant resilience capabilities.
Resilience elsewhere within resilience spectrum
This paper has not specifically investigated resilience standards for those events which are not frequent but are also not extremely infrequent. Such events generally include some demand reduction requirement to allow the system to maintain supply. The most common example is probably the first stage water restrictions applied during droughts. Killen, 2019, advised that those restrictions are commonly applied at about a 1 in 10 and 1 in 20-year frequency in Australia.
Some water authorities have adopted strategies which provide for water restriction notices being issued for non-drought related events which have recurrences of greater than, say, 100 years. Examples include flood, raw water high turbidity and critical infrastructure failure events. Existing level of service standards related to flood protection could be leveraged to determine such frequencies.
It is necessary to have a pre-known trigger and particularly so for non-drought events which can occur without warning. For droughts it is commonly a dam low water level being reached. For non-drought resilience, the restriction application trigger might be a turbidity or water production level.
Tools such as the Tank Sizing Tool and the Resilience Model are useful for investigating the demand reduction needed to allow a system to maintain supply while impacted by a resilience event.
Conclusion
The intent of this discussion paper is to identify existing standards which could be adopted to establish how much water supply resilience is enough, and to discuss strategies for providing adequate resilience measures. To that end, the two ends of the resilience spectrum, i.e., relatively frequently occurring, and exceptionally infrequent events have been investigated.
Water supply levels of service of 10 to 15 years are outlined in the Hunter Water version of the Water Supply Code of Australia. Interruption rates collated by BOM indicate that Australian water supply systems are generally operating at similar levels of service. These levels of service can be used for frequently occurring supply event disruptions. Storage tanks are the main source of resilience for many water supply areas for such events. The storage volumes needed to meet resilience requirements can be determined using these levels of service in conjunction with the quantitative resilience methods which are more effective than previously used qualitative methods.
Emergency risk guidelines such as the National Emergency Risk Assessment Guidelines and the Queensland Emergency Risk Management Framework provide guidance for the level of service which should be required for the provision of water supplies in the event of exceptionally infrequent events. A level of service between 1,000 and 10,000 years is specified. High-level assessments of Queensland’s water supply schemes amended to include interconnected independent sources and water treatment facilities suggest that a resilience standard based on EMSV emergency supplies is workable and would provide for all types of resilience.
The Author
Lee Donaldson
Lee has been investigating water system resilience and the role of storage tanks in distribution systems for several years. His investigations have focused on the stochastic nature of water supply demands and the probabilities of concurrent failure events and how water supply systems, and in particular storage tanks interact with such events.
He has written five related papers for the AWA e-Journal. He has also developed tools for optimizing storage tank volumes and other distribution system infrastructure items while maintaining supply during maximum day and failure events.
References
BOM, 2022, National Performance Report 2020–21: Urban Water Utilities, part B, Bureau of Meteorology.
Bross et al, 2019, Insecure Security: Emergency Water Supply and Minimum Standards in Countries with a High Supply Reliability, Water, DOI 10.3390/w11040732.
Donaldson, L 2018-1, Water Supply Risk Assessments Using Stochastic Peaking Factors, Water e-Journal, DOI 10.21139/wej.2018.031.
Donaldson, L 2018-2, Sizing Water Distribution Storages for Persistence and Emergency Demands, Water e-Journal, DOI 10.21139/wej.2018.040.
DNRME, July 2019, Water Security for South East Queensland, Position Paper on the Review of the Desired Level of Service Objectives, Department of Natural Resources, Mines and Energy, State of Queensland.
Killen, A 2019, Water Security Levels of Service Reviewing Concept and Development in South East Queensland, Water e-Journal, DOI 10.21139/wej.2019.006.
NERAG, 2015, National Emergency Risk Assessment Guidelines, Australian Institute for Disaster Resilience.
QERMF, 2015, Queensland Emergency Risk Management Framework, Queensland Fire and Emergency Services.
US EPA, 2011, Planning for an Emergency Drinking Water Supply, U.S. Environmental Protection Agency’s National Homeland Security Research Center.
Wilson B, 2023, Solving the Water Quality Vs Service Reliability Challenge, Poster Paper, Ozwater’23.
WSAA, 2009, Water Supply Code of Australia WSA 03—2002-2.3, Hunter Water Edition Version 1.
WSAA, 2011, WSA 03-2011 Water Supply Code of Australia Version 3.1.