Advanced optimisation in pilot-scale trial and emergency pre-treatment design to produce safe drinking water
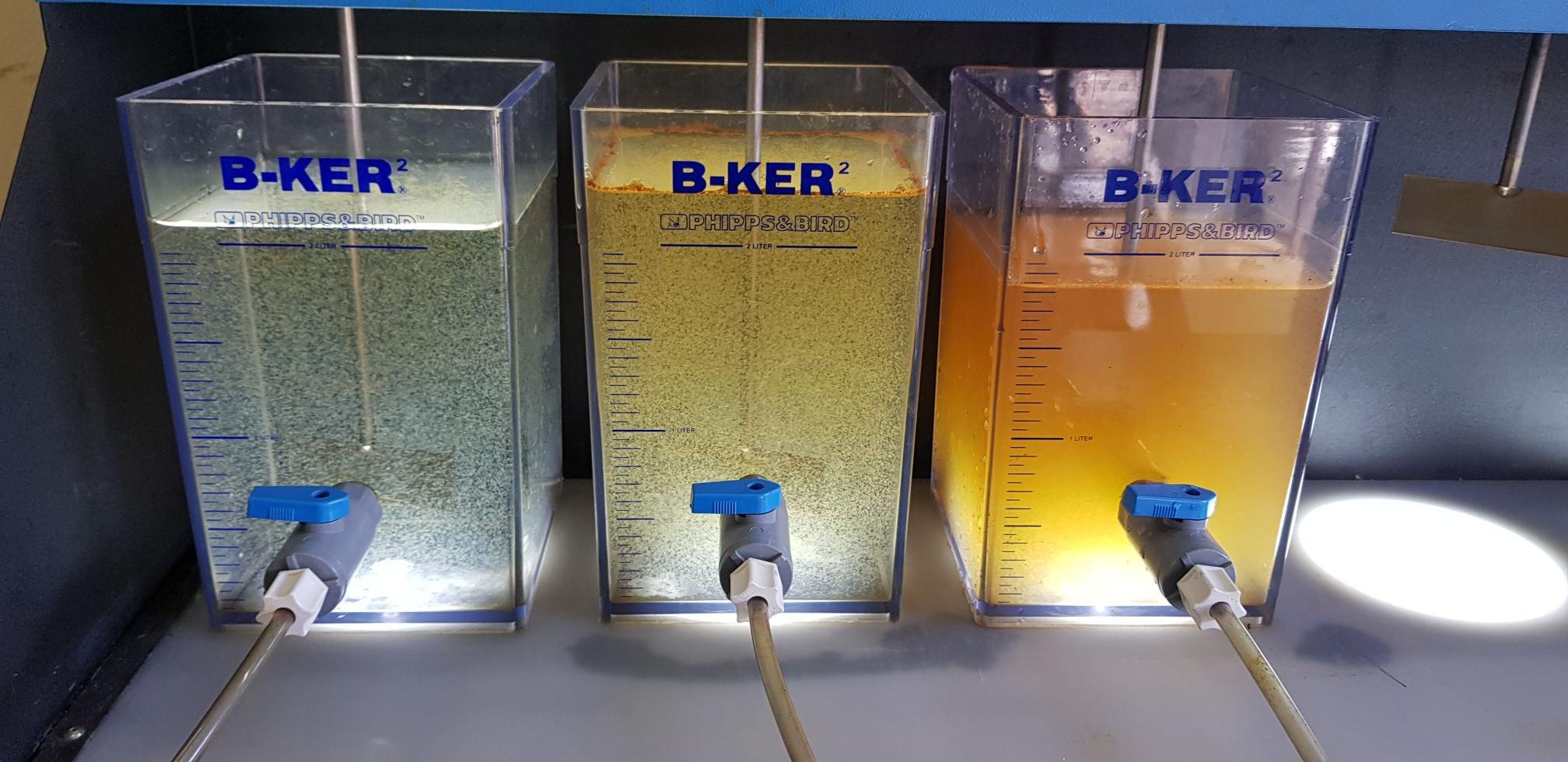
DOWNLOAD THE PAPER
Abstract
Following severe drought in 2018-19, bushfires in late 2019, and three successive extreme rain events between 2020 and 2022, the water quality in Warragamba Dam, the largest water source in Sydney, has significantly deteriorated. This resulted in a turbidity of >110 NTU and a true colour of >100 CU420, representing a significant increase of suspended particles and natural organic matter respectively in raw water, after heavy rainfall in July 2022. Through advanced testing and optimisation at the pilot-scale contact filtration plant and designing an emergency pre-treatment strategy, Sydney Water was able to produce safe drinking water (turbidity <0.20 NTU) from full-scale Water Filtration Plants (WFPs) that have contact filtration technology, to avoid potential severe impacts on 85% of Sydney’s water supply and ensure drinking water met Australian Drinking Water Guidelines, while water quality remained outside the design envelope of the plants. The novel optimisation in a pilot-scale contact filtration plant for increased suspended particles (turbidity) and natural organic matter (true colour) in raw water caused by extreme events is discussed in this paper. The increase of true colour and dissolved organic carbon (DOC) represents a significant increase of natural organic matter (NOM) in the raw water due to extreme events. The characterisation of DOC of raw water and its impact on the contact filtration water treatment process is also discussed in this paper.
Introduction and context
Sydney’s Warragamba Dam is the largest water source with a storage capacity of over 2,000 gigalitres (billion litres) of water. The Warragamba Dam supplies raw water to the three Water Filtration Plants (WFPs) and, after treatment, these WFPs produce safe drinking water for more than 4 million people in Sydney. These three WFPs downstream of the Warragamba Dam are contact filtration treatment plants. Hence, the designed raw water quality envelope for these WFPs is low, with turbidity <7 NTU and true colour <30 CU420 (colour unit measured at 420 nm). The Warragamba catchment experienced a severe drought in 2018-19 and significant bushfires in late 2019. Following the extreme drought and bushfires, the drought-breaking heavy rain fell in the catchment in February 2020 and increased the dam level from 43% (870 gigalitres) to 74% (1500 gigalitres) within five days. A second extreme rain event occurred in March 2021 when the total inflow into the dam was 1500 gigalitres of water in five days. A third significant amount of rain fell in the dam’s catchment in early July 2022. From a five-day rain event in July 2022, the peak inflow to the dam was more than 550 gigalitres of water on a single day, which was close to the city’s water demand for a whole year. Due to the successive extreme rain events, following severe drought and bushfires enhanced by climate change, the water quality in the dam has significantly deteriorated. Turbidity of >110 NTU, true colour >100 CU420, and dissolved organic carbon (DOC) >13 mg/L were observed in the dam after the third major rain event in July 2022. While the increased turbidity represents an increase of suspended particles, the increased true colour and DOC represent a significant increase of natural organic matter in the raw water caused by extreme events. Subsequently, the best water quality at the top of the dam was turbidity 30 NTU, true colour 75 CU420, and DOC 8.5 mg/L, which was the only source of water available for the WFPs but was well above the designed water quality envelope of these contact filtration plants. The increased turbidity (suspended particles) in raw water causes rapid blockage in filters in contact filtration that triggers frequent backwashes of filters due to headloss. The increased concentration of natural organic matter (elevated true colour and DOC) in raw water results in ‘weak floc’ formation during coagulation which causes filter turbidity breakthroughs due to flocs breaking up within the filter bed and triggering filter backwashes due to turbidity spikes. As a consequence, both elevated turbidity and natural organic matter (true colour, DOC) in raw water results in short filter run time and significant reduction of plant production rate. Therefore, it was a very high risk to supply safe drinking water due to the existing contact filtration plants not being designed to be able to treat the raw water.
Experiments
Contact filtration jar tests for deteriorated quality source water
An advanced bench-scale jar tests and monitoring plan were developed for the deteriorated source water from Warragamba Dam (Figure 1). The jar test procedure matched the contact filtration process of existing WFPs at Sydney Water, using lime to adjust alkalinity and pH, pre-chlorination, and then ferric chloride as primary coagulant, cationic polyDADMAC as secondary coagulant followed by polyacrylamide dosing to enhance floc formation, and then filtration by using filter paper. The advanced jar test method enabled to proper evaluation of ‘floc characteristics’ that includes assessment of flocs size, flocs compactness/ fluffiness, water clarity around flocs, flocs settling rates, and filtered water quality (Figure 2). The objective of the series of jar tests was to find the best combination of metal coagulant (ferric chloride) and polymer to improve the floc characteristics for the deteriorated quality raw water. The aim of the jar tests was to find ‘smaller flocs’ to reduce solid loading to filters and reduce headloss in contact filtration while raw water turbidity is very high, and ‘stronger flocs’ to reduce filter turbidity breakthrough in contact filtration which generally occurs due to elevated natural organic matter (true colour and DOC) in raw water.
Figure 1: Sydney Water’s optimisation plan for contact filtration and test plan for emergency pre-treatment process to improve treatment of significantly deteriorated source water from Warragamba Dam
Figure 2: Jar tests and evaluation of floc characteristics
Pilot-scale contact filtration plant trials
A pilot-scale contact filtration plant was used for novel optimisation of contact filtration water treatment for the significantly deteriorated quality of raw water. A truck delivered the poor-quality water from the dam to the pilot plant. The pilot filtration plant mimicked the full-scale WFP’s contact filtration process. The optimised dose combination of metal coagulant (ferric chloride) and polymer from the jar tests was applied at the pilot plant. The pilot-scale contact filtration trial enabled the assessment of ‘solid loading’ to the filter and head-loss build-up and, ‘floc chacarteristics’ and filter turbidity breakthrough (Figure 3). The chemicals combination was then further optimised at the pilot plant to reduce solid loading and filter headloss, and improve ‘floc strength’ to reduce turbidity breakthrough. The aim of the pilot plant trial was to increase filter run time and plant production rate by reducing headloss and turbidity breakthroughs.
Figure 3: Evaluation of ‘floc characteristics’ in pilot filter columns to improve filtration performance and plant production capacity by reducing headloss (solid loading) and turbidity breakthroughs.
Optimisation of the full-scale contact filtration process of the Water Filtration Plants (WFPs)
The jar tests and pilot-scale contact filtration trial data were then applied at the full-scale Orchard Hills WFP to optimise chemicals for the treatment of deteriorated quality raw water. The filter performance of full-scale Orchard Hills WFP was closely monitored for the raw water treatment above the design limit of the WFP and compared with pilot-scale trial data. Filters ripening profile, run time, turbidity breakthroughs, turbidity profile during filter run, and head-loss were monitored and analysed at Orchard Hills WFP.
Jar tests and water quality analysis for emergency pre-treatment process
A comprehensive test plan (Figure 1) was developed to carry out jar tests to design emergency pre-treatment processes at the WFPs. The emergency pre-treatment processes included, a chemical-assisted settling process by dosing ferric chloride in Prospect Reservoir for pre-treatment for Prospect WFP, a conventional sedimentation process by re-commissioning an old disused sedimentation tank for pre-treatment for Orchard Hills WFP, and a high-rate ballasted sedimentation process (ActifloTM) for pre-treatment for Warragamba WFP. A monitoring plan was developed to analyse the settled water from Jar tests for each pre-treatment processes. Based on the jar tests, emergency planning works were conducted for the design, construction, and commissioning of the pre-treatment process units for treating raw water through chemical-assisted settling, conventional sedimentation, or high-rate clarification. The objective of the emergency pre-treatment was to improve the water quality before contact filtration at the WFPs.
DOC removal by treatment processes and organic characterisation of water
Several jar tests were conducted for the Warragamba Dam raw water samples to assess the DOC removal performance by various treatment processes - contact filtration of existing WFPs, conventional sedimentation for emergency pre-treatment at Orchard Hills WFP and high-rate ballasted sedimentation process for emergency pre-treatment at Warragamba WFP. Jar tests for the ‘contact filtration’ process applied coagulation by ferric chloride and polyDADMAC doses followed by filtration in filter paper without any settling steps mimicking full-scale WFPs, the ‘conventional sedimentation’ process applied flocculation and settling time after coagulation mimicking Orchard Hills old sedimentation process, and the ‘ballasted sedimentation’ process applied micro sand (ballast material) and polyacrylamide dose in flocculation step and settling time mimicking the emergency Actiflo design at Warragamba WFP.
A comprehensive test plan was developed to carry out the organic characterisation of raw water, settled water from various pre-treatment processes from jar tests, and filtered water from the contact filtration pilot plant. The objectives of organic characterisation of water samples were to identify the dominant fractions at elevated DOC of raw water and its relationship with ‘weak floc’ formation which impacts filter performance by turbidity breakthroughs, and removal of DOC fractions by various treatment processes.
The organic characterisation of the water samples was performed at the Australian Water Quality Centre (AWQC) laboratory in South Australia. The tests included rapid NOM fractionation and determining the concentration of DOC fractions in mg/L and % of DOC into four different fractions – Very hydrophobic acid (VHA), slightly hydrophobic acid (SHA), charged hydrophilic acid (CHA) and neutral fractions (NEU). Rapid NOM Fractionation at AWQC was based on the selective adsorption of DOC fractions to a series of ion exchange resins. The VHA fraction is known as Humic acids, which has a high molecular weight, and is a highly coloured fraction. VHA was determined by adsorption on DAX-8 resin. SHA is known as Fulvic acids, which has a moderate molecular weight and is a less coloured fraction. SHA was determined by adsorption on XAD-4 resin. CHA was determined by adsorption on IRA-958 resin. CHA is of higher specific charge and high specific UV absorbance fraction. NEU fraction is the smaller molecular weight, uncharged and uncoloured fraction and it has low specific UV absorbance. NEU fraction was determined by non-absorbance in any resin.
Experiments
Water Quality Analysis
Several raw water samples were collected from the Warragamba Dam and at the inlet of Orchard Hills Water Filtration Plant (WFP) for detailed water quality analysis. Based on the grab sample analysis, the July 2022 major rain event in the Warragamba catchment is represented by a significant increase in turbidity of 73 NTU, true colour of 92.9 CU420, UV254 absorption of 0.34 /cm, DOC of 9 mg/L and TOC of 10.3 mg/L (Table 1). Data analysis revealed that the increase of true colour in Warragamba Dam is strongly correlated with the increase of DOC in raw water and a linear correlation exists between these two parameters which can be expressed by the equation below (Figure 4).
True colour 420 = DOC × 11.57 – 15.229
Therefore, while the increased level of turbidity represents an increased level of suspended solids, the elevated true colour, DOC, and UV254 absorption represent an increased concentration of natural organic matter in the raw water after July 2022 rain event. It was also identified from the data analysis that, the TOC and DOC of Warragamba Dam Water are linearly correlated and that, most of the organics are in a dissolved state in DOC, rather than in a particulate form in TOC (Figure 5).
Table 1: Warragamba raw water quality analysis following the rain event of July 2022
Figure 4: True colour and Dissolved Organic Carbon (DOC) relationship, Warragamba Dam water, July-August 2022
Figure 5: Total Organic Carbon (TOC) and Dissolved Organic Carbon (DOC) relationship, Warragamba Dam Water, July-August 2022
Jar tests, contact filtration pilot plant trials and advanced optimisation at the Water Filtration Plants
A total of 60 jar tests and 40 pilot-scale contact filtration trials were conducted over a period of three months. A range of poor-quality raw water was tested in the jar tests and pilot plant trials, with maximum turbidity of 77.6 NTU and maximum true colour of 103 CU420 (Table 2). The optimum dose rates of coagulants (12-17 mg/L Ferric chloride as FeCl3 and 3.5-4.5 mg/L polyDADMAC) were identified from jar tests for the treatment of raw water with turbidity >70 NTU representing a higher concentration of suspended solids, and true colour >100 CU420 representing significantly increased concentration of natural organic matter in the water (Table 2). The ‘floc characteristics’ were assessed in the jar tests and the optimum combination of coagulant doses was identified based on ‘smaller flocs’ to reduce solids loading in filters and thereby reduce head loss build-up while raw water contains higher turbidity, and ‘compact flocs’ with better settling rate in jars representing ‘stronger flocs’ to reduce filter turbidity breakthrough due to higher concentration of natural organic matter (true colour) in raw water.
Table 2: Optimal chemical dose identified from 60 Jar tests and 40 Pilot filtration plant runs
The optimum dose rates from jar tests were then applied in the pilot-scale contact filtration plant to assess the pilot filter performance mimicking the full-scale contact filtration WFPs in Sydney Water. Pilot plant trials enabled the assessment of detailed ‘floc characteristics’ in the filter column including flocs size, flocs concentration /solids accumulation on the top of filter bed, fluffiness or compactness of flocs, flocs strength and any flocs break-up while passing through filter bed and turbidity breakthrough (Figure 3). Pilot plant ‘filter performance’ data was assessed for filtered water quality, filter run time, filter head loss build-up, filtered water turbidity profile during the filter run, and production capacity of the filter. Pilot plant trials successfully discovered the impact of coagulation pH and coagulant dose rates on ‘floc characteristics’ and ‘filter performance’. As a result of a series of pilot plant optimisation runs, a novel combination of coagulation pH 8.5, ferric chloride dose rate of 10-16 mg/L as FeCl3, and cationic polymer dose rate of 3.5-3.7 mg/L as polyDADMAC (Table 2) were identified for treatment of raw water in the contact filtration plant, while this raw water quality was beyond the design limit of contact filtration (Table 2). With this novel optimisation, the contact filtration pilot plant trial demonstrated safe drinking water production with turbidity <0.1 NTU, true colour <10 CU420, and a good filter run time of 26-48 hours (Table 2) representing reduced head-loss build-up by selecting ‘smaller flocs’ and reduced turbidity breakthroughs by generating ‘stronger flocs’ with optimum combination of coagulant doses.
From the series of contact filtration pilot plant trials, it was identified that, with an optimum dose rate of cationic polyDADMAC, careful and gradual reduction of ferric chloride dose produced smaller flocs, reduced solid loading to the filter (Figure 3), and subsequently, it delayed filter turbidity breakthrough (Figure 6), and increased run time.
Figure 6: Pilot plant trial #21 - Reduced ferric chloride dose rate delayed turbidity breakthrough and
increased filter run time, with filtered water turbidity <0.10 NTU
It was also identified from the contact filtration pilot plant trials that, for raw water turbidity of 50-73 NTU and true colour 79 to 92 CU420, with an optimum ferric chloride dose rate of 17 mg/L as FeCl3, the increase of cationic polymer (polyDADMAC) dose increased the removal of DOC and true colour but reduced the filter run time (Table 3) due to turbidity breakthrough. Therefore, for the increased natural organic matter (true colour and DOC) in raw water, an optimum dose rate of 3.5 – 3.8 mg/L polyDADMAC was identified achieving ‘stronger flocs’ that reduce filter turbidity breakthroughs.
Table 3: Impact of increased cationic polyDADMAC dose on filtered water quality and filter run time
The contact filtration pilot plant trials concluded that, for the tested raw water with turbidity up to 70 NTU and true colour up to 93 CU420, the low pH coagulation at 6.5, increased ferric chloride dose above 17 mg/L, or increased cationic polyDADMAC dose above 4.5 mg/L, results ‘weak floc’ formation which caused turbidity breakthroughs in filters, resulting in significantly shorter filter run time and reduction in plant production rate. This confirms that to produce safe drinking water from a contact filtration plant, accurate optimisation of chemicals would be required, targeting ‘smaller flocs’ for less solid (flocs) loading and head-loss build-up in the filter, and ‘stronger flocs’ to reduce turbidity breakthrough, and thus filter run time and production rate can be increased.
Pilot plant trial data of optimum chemical dose combination was then applied at the full-scale Orchard Hills WFP. It resulted in safe drinking water production from the full-scale contact filtration plant with filtered water turbidity profile <0.10 NTU during filter run (Figure 7) and true colour <10 CU420, by treating raw water of 30 NTU and true colour 75 CU420, which were above the design limit of the plant.
Implementation of the optimum dose rates combination of coagulants identified from jar tests and pilot-scale contact filtration trial for raw water with higher turbidity (suspended solids) and natural organic matter (true colour and DOC) resulted in ‘smaller flocs’ and ‘stronger flocs’ in the full-scale plant at Orchard Hills WFP and thereby reduced head-loss build up and turbidity breakthroughs in full-scale filters at the plant. As a result, at Orchard Hills WFP the filters were operated with longer run time, and the plant production rate was increased to meet demand.
Figure 7: SCADA turbidity trends of all 10 dual media filters (DMFs) at Orchard Hills WFP producing safe drinking water with turbidity <0.10 NTU, improved turbidity profile during filter run, reduced turbidity breakthroughs, by advanced optimisation for significantly deteriorated raw water
Emergency pre-treatment design for WFPs
A series of jar tests were carried out to design emergency pre-treatment of raw water to improve the water quality before contact filtration at the WFPs. The projects included an emergency chemical-assisted settling process by dosing ferric chloride in Prospect Reservoir for pre-treatment of 500 ML/d raw water for Prospect WFP, refurbishment and re-commissioning of an old sedimentation tank for pre-treatment of 60 ML/d raw water by conventional sedimentation (dosing ferric chloride, cationic polyDADMAC and non-ionic polyacrylamide) for Orchard Hills WFP and, design, construction, and commissioning of a high-rate lamella clarifier (Ballasted sedimentation by ActifloTM) for pre-treatment (dosing ferric chloride, cationic polyDADMAC, microsand and polyacrylamide) of 1.0 ML/d raw water for Warragamba WFP.
The jar tests and pilot-scale settling tests for emergency pre-treatment design by chemical-assisted settling in Prospect Reservoir resulted in the removal of 95% of turbidity and 90% of true colour from the raw water turbidity >110 NTU and true colour >100 CU420 (Table 4).
Table 4: Emergency chemical-assisted settling test results for pre-treatment for Prospect WFP
The jar tests for conventional sedimentation for Orchard Hills WFP identified that, with an optimum dose of 20 mg/L Ferric Chloride as FeCl3 and 2 mg/L cationic polymer as polyDADMAC doses, the settled water turbidity of 5.5 NTU, true colour of 14.99 CU420 and UV254 absorption <0.1 /cm can be achieved (Table 5). Therefore, pre-treatment by conventional sedimentation at Orchard Hills WFP accomplishes as much as 73% reduction of turbidity, 72% reduction of true colour and 52% reduction of UV254 absorption from poor quality raw water of turbidity 20.9 NTU and true colour 53 CU420 (Table 5).
Table 5: Conventional sedimentation process jar tests for emergency pre-treatment of raw water at Orchard
Hills WFP
Table 6: Ballasted sedimentation (ActifloTM) process jar tests for emergency
pre-treatment of raw water at Warragamba WFP
The jar tests for high-rate ballasted sedimentation for pre-treatment for Warragamba WFP demonstrated that, with an optimum dose of 10 mg/L Ferric Chloride as FeCl3 and 2 mg/L cationic polymer as polyDADMAC doses, the settled water turbidity <1 NTU, true colour <20 CU420 and UV254 absorption of 0.116 /cm can be achieved (Table 6). Therefore, pre-treatment by ballasted sedimentation at Warragamba WFP can lead to > 95% removal of turbidity, >60% removal of true colour and >40% removal of UV254 absorption from poor-quality raw water of turbidity 20.9 NTU and true colour 53 CU420 (Table 6).
Raw water organic characterisation and removal by treatment processes
In total, ten samples were collected from Warragamba Dam following the major rainfall event in July 2022 for detailed organic characterisation. The samples contained very high DOC, mostly in the range of 7.1 to 8.1 mg/L and a minimum of 5.9 mg/L. The DOC fractions were as follows: very hydrophobic acid (VHA) fractions 4.5 to 6.3 mg/L, slightly hydrophobic acid (SHA) fractions 0.8 to 1.2 mg/L, charged hydrophilic (CHA) 0.1 to 0.4 mg/L and neutral hydrophilic (NEU) 0.3 to 0.7 mg/L (Figure 8).
Figure 8: Dissolved Organic Carbon (DOC) and its fractions, Warragamba Dam water, July - August 2022
Data analysis for DOC and its fractions revealed that, with the increase of DOC in Warragamba dam raw water, predominantly the VHA fractions rose. A linear correlation exists between the increase of DOC and VHA fractions which can be expressed by the equation below. The increase of other fractions, namely SHA, CHA and NEU, is insignificant.
VHA Warragamba = DOC Warragamba × 0.7564 + 0.042
Results from jar tests for contact filtration, conventional sedimentation and high-rate ballasted sedimentation processes showed that for the raw water DOC in the range of 7 to 8 mg/L, all three treatment processes achieved similar DOC removals in the range of 20-30% of raw water DOC. This resulted in a treated water DOC in the range of 5 to 5.5 mg/L range (Figure 9). Like the DOC removal in all three treatment processes, the removal of VHA fractions is also consistently 20-30% of the raw water by contact filtration, conventional sedimentation, and ballasted sedimentation processes (Figure 10). The SHA fractions removal is between 11% and 22% of raw water by all three treatment processes (Figure 10).
Figure 9: Dissolved Organic Carbon (DOC) and its removal by various treatment processes
Figure 10: Removal of VHA and SHA fractions by various treatment processes
Due to the similar DOC, and its fractions (VHA and SHA) removal by all three treatment processes, the composition of DOC in treated water remained similar in all processes. The treated water contained a predominant fraction, the VHA in the range of 70.4% to 73.9% of DOC, and the second largest fraction, the SHA in the range of 15.2% to 16.9% of DOC. In the minor fractions, the CHA was in the range of 2.2% to 5.2% of DOC and the NEU was in the range of 6.9% to 10.9% of DOC (Figure 11).
Figure 11: Fractions of dissolved organic carbon (DOC) in raw water and treated water
In total, nine pilot-scale contact filtrations trial runs were analysed for contact filtration performance with variable DOC and its fractions. Results revealed that, for the raw water DOC <7 mg/L, the pilot contact filters’ run time was around 40 hours, which indicates an increased ‘floc strength’ and reduced turbidity breakthroughs in filters. For the raw water DOC >7.5 mg/L, the pilot filters’ run time was significantly shorter in the range of 20-30 hours, representing that the filters experienced turbidity breakthroughs leading to early backwashes due to ‘weak flocs’ formation with increased concentration of natural organic matter (DOC) in raw water (Figure 12). The analysis also found that, like the relationship of DOC with filter run time, the increase in VHA fractions is strongly correlated to reduced filter run time (Figure 13) in the contact filtration process.
Figure 12: Contact Filtration Pilot Filter Run time relationship with DOC of raw water
Figure 13: Contact Filtration Pilot Filter Run time relationship with VHA fraction of DOC of raw water
Conclusion
Sydney Water was able to produce safe drinking water for customers which complied with the Australian Drinking Water Guidelines. This was possible by conducting advanced bench-scale jar tests, novel optimisation at the pilot-scale contact filtration plant and, optimisation at the full-scale WFPs for extremely deteriorated quality raw water from the Warragamba Dam. Sydney Water’s extensive jar tests to design emergency pre-treatment processes and their design, construction, and commissioning, prepared the WFPs to undertake successful water treatment in the event of a further deterioration of raw water at the inlet to the plants. The organic characterisation of raw water with elevated natural organic matter from extreme rain events in the catchment and its relationship with treatment performance presented in this paper fills up the knowledge gap. Through this work, Sydney Water managed a potentially serious outcome for customers and ensured drinking water met Australian Drinking Water Guidelines, despite significant degradation of source water quality caused by three successive major rain events in the last three years.
Acknowledgement
The authors acknowledge the cooperation from Sydney Water’s internal teams and WaterNSW during the jar tests, pilot plant trial, and optimisations.
The Authors
ASM Mohiuddin
Process Integration Manager, Sydney Water
ASM Mohiuddin holds a Bachelor of Science degree in Chemical Engineering and a Master of Engineering degree in Water, Wastewater and Waste. Mohiuddin has over 20 years of process engineering experience in the industry. He currently works as a Process Integration Manager in Water Supply & Production at Sydney Water and manages process engineering and capital interface teams. He leads drinking water improvement projects, optimisation, R&D trials, and selection of treatment technologies during water treatment upgrades.
Yue Cong Wang
Senior Process Engineer and Modelling Specialist, Sydney Water
Yue Cong Wang is a Senior Process Engineer and Modelling Specialist at Sydney Water. He has over twenty-eight years of numerical modelling and model development experience gained through practical and research activities in water and wastewater fields. He also has very strong computer programming skills and has developed several customised software for data manipulation, management, and presentation in support of hydraulic and environmental modelling activities, as well as process modelling for water filtration plants.
Saravanamuthu Vigneswaran
Emeritus Professor, University of Technology Sydney
Prof. S. Vigneswaran is currently an Emeritus Professor at the University of Technology, Sydney (UTS) a Distinguished Fellow of the International Water Association (IWA). He was Distinguished Professor of Environmental Engineering in the Faculty of Engineering and IT at the University of Technology, Sydney (UTS) till December 2021. From 2008 to Feb 2018, he served as the Inaugural Director of UTS’s Centre of Technologies in Water and Wastewater (CTWW).
References
1. Mohiuddin, A., Wang, Y., Gabus, C., Lingat, P. 2022. Identifying alternative treatment options for removal of bushfire-related contaminants. Water e-Journal, Journal of Australian Water Association, 8 (1).
2. Mohiuddin, A., Rajanayagam, C., Kearney, C. 2014. Optimisation of non-ionic polymer to address production issues with high-colour low -turbidity raw water. Water, Journal of Australian Water Association, 41 (8), 58-63.
3. Mohiuddin, A., Wang, Y. 2017. Statistical model development to anticipate filter breakthrough and optimise chemicals during high colour raw water events. Water e-Journal, Journal of Australian Water Association, 2 (4).
4. Mohiuddin, A., Cox, P., Blayney, B. 2020. Impact of the Millennium Drought on water filtration plants. Water e-Journal, Journal of Australian Water Association, 5 (1).